Chapter 1. Frequency Conversion of Laser Crystals
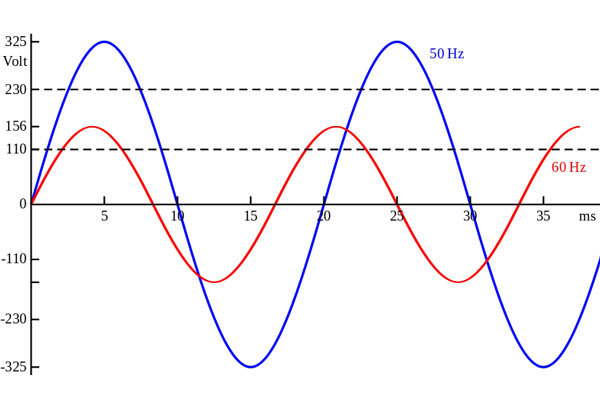
Frequency conversion is the process of changing the frequency, wavelength, or polarization of a laser beam using nonlinear optical materials. This chapter will discuss the different frequency conversion processes, including harmonic generation, sum frequency mixing, and difference frequency mixing. We will also compare the frequency conversion properties of Ti Sapphire Crystal and other laser crystals.
1.1 Harmonic Generation
Harmonic generation uses a nonlinear optical material to convert a laser beam at a fundamental frequency (ω) into a higher harmonic frequency (2ω, 3ω, etc.). The most common nonlinear optical materials used for harmonic generation are KTP and LBO.
The conversion efficiency of harmonic generation depends on several factors, including the power, wavelength, and polarization of the fundamental beam, as well as the crystal orientation and temperature of the nonlinear optical material. Ti Sapphire Crystal is a popular laser crystal for a harmonic generation due to its broad tuning range and high gain.
1.2 Sum Frequency Mixing
Sum frequency mixing is the process of combining two laser beams at different frequencies (ω1 and ω2) to generate a new beam at a frequency equal to the sum of the two frequencies (ω1+ω2). The most common nonlinear optical material used for sum frequency mixing is LBO.
The conversion efficiency of sum frequency mixing depends on several factors, including the power, wavelength, and polarization of the two input beams and the crystal orientation and temperature of the nonlinear optical material. Ti Sapphire Crystal is a popular laser crystal for sum frequency mixing due to its broad tuning range and high gain.
1.3 Difference Frequency Mixing
Difference frequency mixing is the process of combining two laser beams at different frequencies (ω1 and ω2) to generate a new beam at a frequency equal to the difference between the two frequencies (|ω1-ω2|). The most common nonlinear optical material used for different frequency mixing is AgGaS2.
The conversion efficiency of difference frequency mixing depends on several factors, including the power, wavelength, and polarization of the two input beams and the crystal orientation and temperature of the nonlinear optical material. Ti Sapphire Crystal is a popular laser crystal for difference frequency mixing due to its broad tuning range and high gain.
1.4 Comparison of Ti Sapphire Crystal and Other Laser Crystals
Ti Sapphire Crystal has unique frequency conversion properties, making it a popular laser crystal for various applications. Ti Sapphire Crystal has a broad tuning range, high gain, and excellent thermal and mechanical properties compared to other laser crystals. Nd: YAG and Nd: YVO4 is also commonly used for frequency conversion due to their high efficiency and gain. KTP and LBO are popular nonlinear optical materials due to their high nonlinear coefficients.
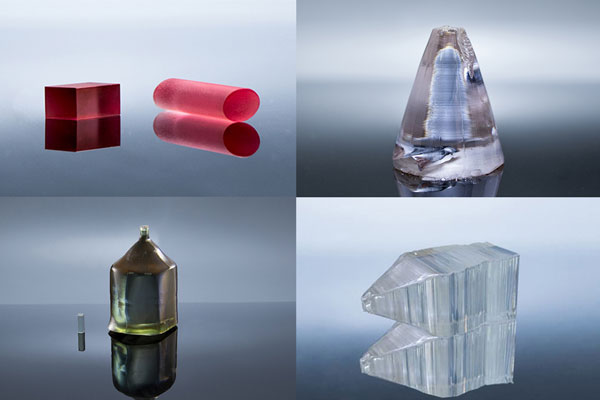
When choosing a laser crystal and nonlinear optical material for a specific frequency conversion application, it is important to consider the desired output wavelength, power, efficiency, and crystal and material properties. Ti Sapphire Crystal and Nd: YAG is versatile laser crystals that can be used for a wide range of frequency conversion applications, while KTP and LBO are commonly used for harmonic generation and sum frequency mixing, and AgGaS2 is commonly used for difference frequency mixing.
1.5 Conclusion
In this chapter, we have discussed the frequency conversion of laser crystals and the different frequency conversion processes, including harmonic generation, sum frequency mixing, and difference frequency mixing. We have also compared the frequency conversion properties of Ti Sapphire Crystal and other laser crystals. Frequency conversion is a powerful tool for generating laser beams at different frequencies, wavelengths, and polarizations and plays a critical role in many laser applications. The choice of laser crystal and nonlinear optical material depends on the specific application requirements, such as the desired output power, wavelength, and efficiency.
Chapter 2. Applications of Laser Crystals
Laser crystals are used in various applications, including material processing, spectroscopy, medicine, and telecommunications. In this chapter, we will discuss some of the most common applications of laser crystals and the advantages of using specific laser crystals for these applications.
2.1 Material Processing
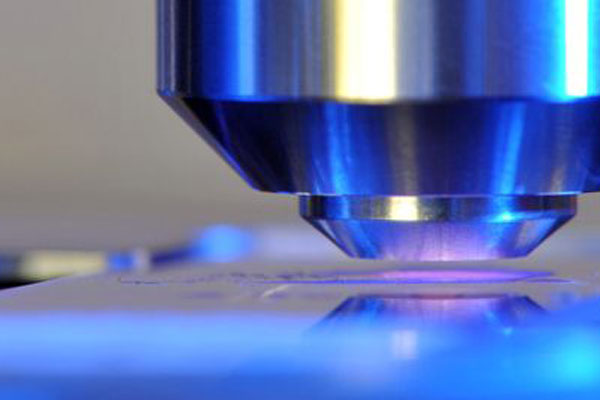
Laser material processing is a widely used application of laser crystals that involves using lasers to cut, weld, drill, and mark various materials, such as metals, ceramics, plastics, and semiconductors. Laser material processing is a versatile and precise method for manufacturing and modifying materials and is commonly used in aerospace, automotive, electronics, and medical devices.
YAG is a popular laser crystal for material processing due to its high efficiency, peak power, and short pulse duration. Ti Sapphire Crystal is also used for material processing due to its broad tuning range and high gain.
2.2 Spectroscopy
Laser spectroscopy is a powerful analytical technique involving lasers to probe the structure and dynamics of atoms and molecules. Laser spectroscopy is used in a wide range of applications, such as chemical analysis, environmental monitoring, and biomedical research.
Ti Sapphire Crystal is a popular laser crystal for spectroscopy due to its broad tuning range and high gain. Other laser crystals used for spectroscopy include Nd: YAG, Nd: YVO4, and Yb: YAG.
2.3 Medicine
Laser medicine is a rapidly growing field that involves using lasers to diagnose and treat various medical conditions, such as cancer, cardiovascular disease, and ophthalmic disorders. Laser medicine is a non-invasive and precise method for treating and diagnosing diseases and has revolutionized many areas of medicine.
Laser medicine is a rapidly growing field that involves using lasers to diagnose and treat various medical conditions, such as cancer, cardiovascular disease, and ophthalmic disorders. Laser medicine is a non-invasive and precise method for treating and diagnosing diseases and has revolutionized many areas of medicine.
Ti Sapphire Crystal is a popular laser crystal for medical applications due to its high pulse energy, high repetition rate, and tunable output wavelength. Other laser crystals used for medical applications include Nd: YAG and Er: YAG.
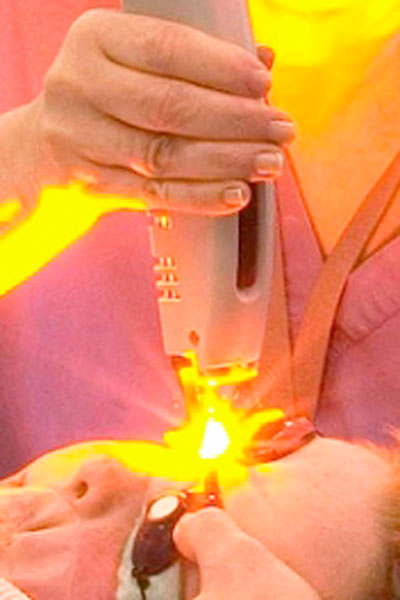
2.4 Telecommunications
Laser telecommunications is a critical component of modern communication systems and involves using lasers to transmit information over long distances through fiber optic cables. Laser telecommunications is a high-speed and reliable method for transmitting information and is used in a wide range of applications, such as the internet, television, and telephony.
Er: Yb: Glass is a popular laser crystal for telecommunications due to its high efficiency and gains at 1550 nm, the standard wavelength used in fiber optic communication systems.
2.5 Conclusion
This chapter has discussed some of the most common applications of laser crystals, including material processing, spectroscopy, medicine, and telecommunications. Laser crystals play a critical role in determining laser performance and efficiency. The choice of laser crystal depends on the specific application requirements, such as the desired output power, wavelength, and efficiency. Laser crystals are a versatile and precise tool for a wide range of applications and have revolutionized many areas of science and technology.
Chapter 3. Advancements in Laser Crystal Technology
The field of laser crystal technology is constantly evolving, driven by advancements in materials science, optics, and electronics. This chapter will discuss recent advancements in laser crystal technology and their impact on laser performance and applications.
3.1 Improved Laser Crystal Growth Techniques
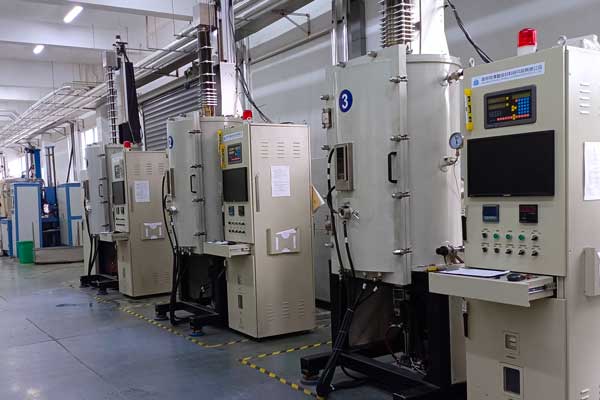
The quality and performance of laser crystals depend on the crystal growth process, which determines the crystal’s composition, defect structure, and homogeneity. Recent advancements in crystal growth techniques, such as the Czochralski method, the Bridgman-Stockbarger method, and the floating zone method, have produced high-quality and large-size laser crystals with improved optical and thermal properties.
For example, the Czochralski method allows for producing large-size single crystals with high optical and thermal homogeneity. In contrast, the Bridgman-Stockbarger method allows for producing multi-component crystals with precise composition control. The floating zone method allows for the production of single crystals with controlled doping and defect structure.
3.2 Novel Laser Crystal Compositions
Recent advancements in materials science have led to the development of novel laser crystal compositions with unique optical and thermal properties. For example, doping Yb ions in a YAG host crystal has resulted in high-efficiency and high-power lasers with improved thermal and mechanical properties. Similarly, doping Ce ions in a YAG host crystal has resulted in scintillation detectors with improved energy resolution and detection efficiency.
Other novel laser crystal compositions include rare-earth doped oxyfluoride crystals, such as GdVO4, which have high gain and efficiency at visible wavelengths, and Tm-doped mixed chalcogenide crystals, which have high gain and efficiency at mid-infrared wavelengths.
3.3 Advanced Laser Crystal Processing Techniques
Recent advancements in laser crystal processing techniques, such as femtosecond laser micromachining and ion implantation, have produced laser crystals with controlled microstructure and optical properties.
For example, femtosecond laser micromachining allows for the fabrication of laser crystals with precise microstructures, such as photonic crystals and waveguides, which can be used for frequency conversion and mode-locking. Ion implantation allows for introduction of controlled defects and dopants in laser crystals, which can modify their optical and thermal properties.
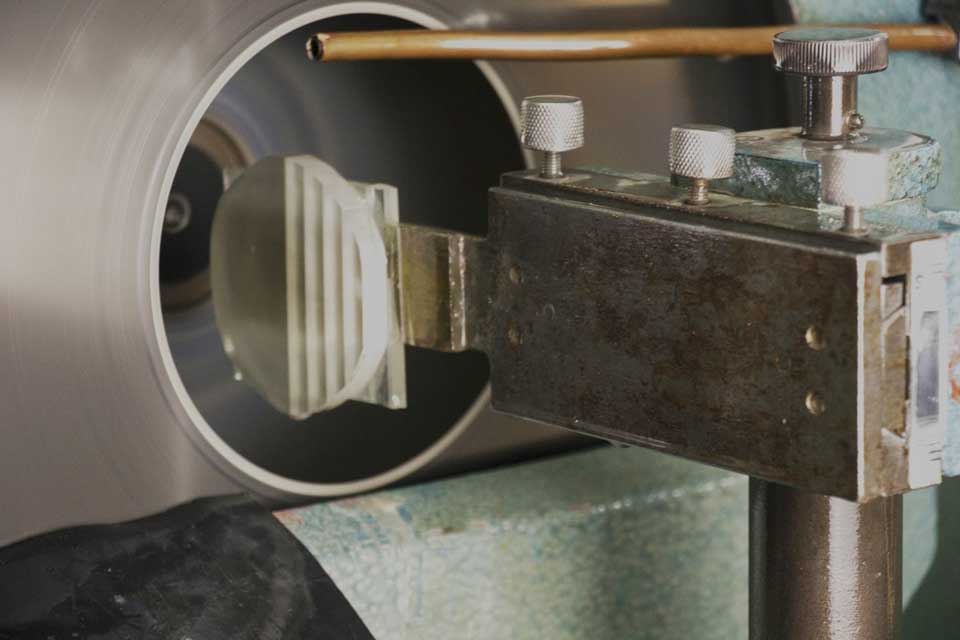
3.4 Emerging Laser Crystal Applications
Advancements in laser crystal technology have enabled the development of emerging applications, such as quantum technologies, high-energy physics, and space exploration.
For example, laser-cooled atomic clocks, based on laser-cooled atoms trapped in a laser crystal, are emerging as the most precise time-keeping devices and have potential applications in navigation, communication, and fundamental physics research. High-power and high-energy laser crystals, such as Yb: YAG and Nd: glass, are used in high-energy physics experiments, such as inertial confinement fusion and particle acceleration. Laser crystals are also used in space-based experiments, such as laser altimetry and communication.
3.5 Conclusion
In this chapter, we have discussed recent advancements in laser crystal technology, including improved crystal growth techniques, novel crystal compositions, advanced crystal processing techniques, and emerging applications. Laser crystal technology is a rapidly evolving field driven by the demand for high-performance and versatile laser systems for various applications. The advancements in laser crystal technology have enabled the development of new and emerging applications and have revolutionized many areas of science and technology.
Chapter 4. Future Directions in Laser Crystal Technology
The field of laser crystal technology is constantly evolving, driven by the demand for high-performance and versatile laser systems for a wide range of applications. This chapter will discuss some of the future directions in laser crystal technology and the potential impact on laser performance and applications.
4.1 Novel Laser Crystal Compositions
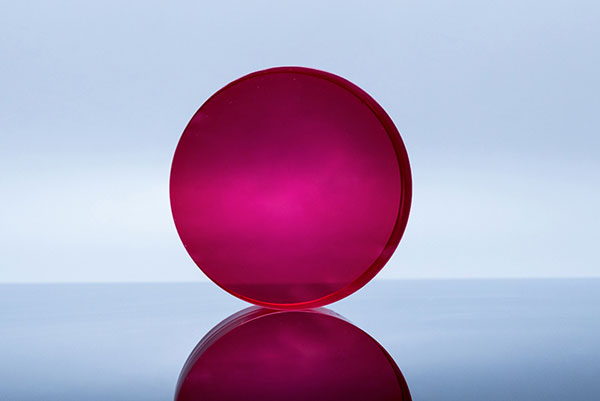
Future developments in laser crystal technology will likely involve discovering and developing novel laser crystal compositions with unique optical and thermal properties. For example, using transition-metal-doped garnet crystals, such as Yb: LuAG and Yb: YAG, has led to high-efficiency and high-power lasers with improved thermal and mechanical properties.
Other promising laser crystal compositions include rare-earth-doped fluoride crystals, such as CaF2 and SrF2, which have high gain and efficiency at ultraviolet and visible wavelengths, and semiconductor-doped laser crystals, such as GaN and GaAs, which have high gain and efficiency at near-infrared and mid-infrared wavelengths.
4.2 Advanced Laser Crystal Fabrication Techniques
Future developments in laser crystal technology will also involve developing advanced fabrication techniques to produce laser crystals with controlled microstructure and optical properties. For example, 3D printing and nanofabrication techniques can produce laser crystals with precise geometries, such as complex photonic structures and waveguides, which can be used for frequency conversion and mode-locking.
Other advanced fabrication techniques include ultrafast laser processing, such as femtosecond laser micromachining and direct laser writing, which can allow for the fabrication of laser crystals with controlled microstructure and optical properties at the micron and sub-micron scale.
4.3 Integrated Laser Crystal Systems
Future developments in laser crystal technology will also involve the integration of laser crystals with other optical and electronic components to form integrated laser systems. For example, integrating laser crystals with microelectromechanical systems (MEMS) and microfluidic devices can allow for the development of compact and versatile laser systems for biomedical and environmental applications.
Other promising integrated laser systems include photonic integrated circuits, which can integrate multiple laser crystals and optical components on a single chip, and hybrid laser systems, which can combine different types of laser crystals and sources for improved performance and versatility.
4.4 Quantum Laser Crystal Technology
Future developments in laser crystal technology will also involve the integration of laser crystals with quantum technologies, such as quantum computing, quantum sensing, and quantum communication. For example, the use of rare-earth-doped crystals, such as Nd: YAG and Yb: YAG, as quantum memory devices have been demonstrated for storing and retrieving quantum information.
Other promising quantum laser crystal technologies include using laser crystals as single-photon sources, quantum entanglement sources, and quantum frequency converters for manipulating and controlling the quantum states of light and matter.
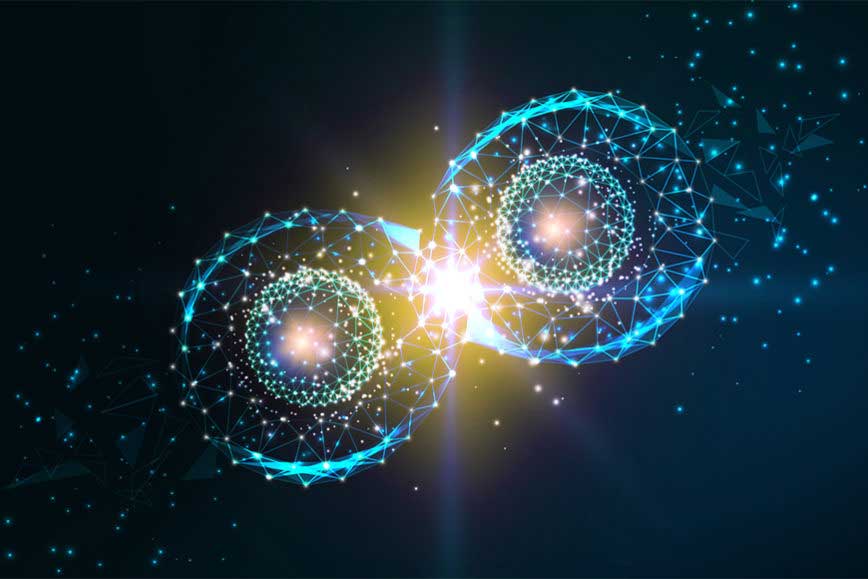
4.5 Conclusion
This chapter discusses future directions in laser technology, including novel laser crystal compositions, advanced fabrication techniques, integrated laser systems, and quantum laser crystal technology. The future of laser crystal technology is promising, with the potential to revolutionize many areas of science and technology, from communication and sensing to quantum technologies and space exploration. The advancements in laser crystal technology will continue to drive the development of new and emerging applications. They will play a critical role in shaping the future of science and technology.
Conclusions
In conclusion, comparing Ti Sapphire crystals with other laser crystals is an important topic in laser physics and technology. Laser crystals play a critical role in determining laser performance and efficiency. The choice of laser crystal depends on the specific application requirements, such as the desired output power, wavelength, and efficiency.
Nd: YAG and Ti Sapphire crystals are popular laser crystals used in various applications, including material processing, spectroscopy, and medicine. YAG is a popular laser crystal for material processing due to its high efficiency, peak power, and short pulse duration. Ti Sapphire crystal is also used for material processing due to its broad tuning range and high gain.
Ti Sapphire crystal is a popular laser crystal for spectroscopy due to its broad tuning range and high gain. Nd: YAG, Nd: YVO4, and Yb: YAG are also used for spectroscopy.
Ti Sapphire crystal is a popular laser crystal for medical applications due to its high pulse energy, high repetition rate, and tunable output wavelength. Nd: YAG and Er: YAG is also used for medical applications.
Er: Yb: Glass is a popular laser crystal for telecommunications due to its high efficiency and gains at 1550 nm, the standard wavelength used in fiber optic communication systems.
The advancements in laser crystal technology have enabled the development of new and emerging applications, such as quantum technologies, high-energy physics, and space exploration. Future developments in laser crystal technology will likely involve discovering and developing novel laser crystal compositions, advanced laser crystal fabrication techniques, integrated laser systems, and quantum laser crystal technology. The field of laser crystal technology is rapidly evolving, driven by the demand for high-performance and versatile laser systems for a wide range of applications. The advancements in laser crystal technology have revolutionized many areas of science and technology and will continue to shape the future of science and technology.
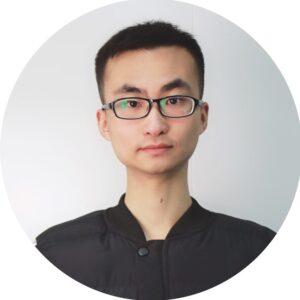
Frank
Frank graduated from the University of Shanghai for Science and Technology, majoring in optics. As a technical engineer at Crylink Company, he deeply understands crystal materials and laser components.
Related Video(s) with this Article
Related Product(s) with this Article
Related Application(s) with this Article