Introduction to Optical Crystals
Optical crystals are unique materials that play a pivotal role in the world of optics and photonics. These crystals have distinct properties that allow them to manipulate light in specific ways, making them invaluable in various technological applications. In this comprehensive guide, we delve deep into the world of optical crystals, exploring their definition, basic characteristics, and the underlying lattice structures that influence their optical properties.
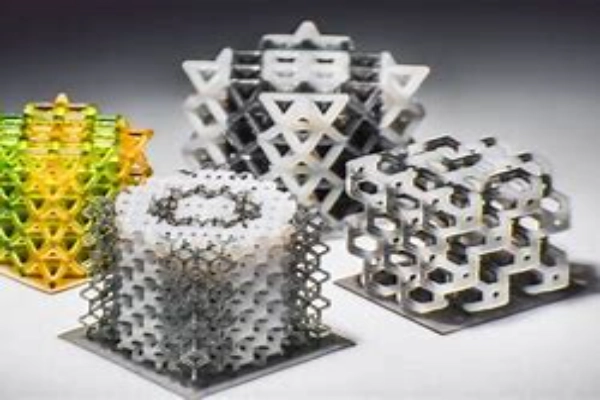
Definition of Optical Crystals
Optical crystals are solid materials in which atoms, ions, or molecules are arranged in a highly ordered, repeating pattern extending in all three spatial dimensions. This order is what gives these crystals their unique ability to interact with light.
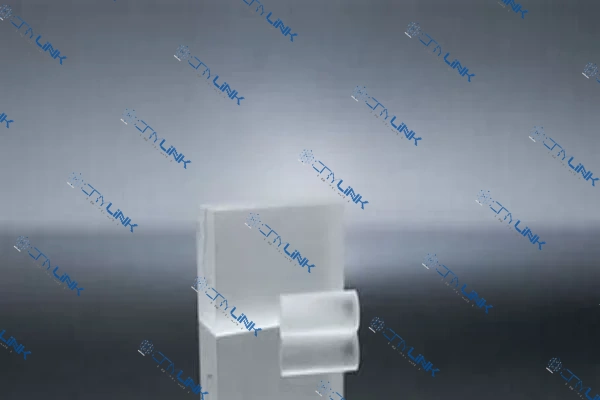
Fundamental Characteristics of Optical Crystals
Optical crystals, with their intricate internal structures and unique properties, have always been at the forefront of scientific exploration, especially in the realm of optics. Among the myriad of characteristics that these crystals possess, three stand out as particularly fundamental: transparency, refractive index, and birefringence. Each of these properties plays a crucial role in determining how optical crystals interact with light, and subsequently, their applications in various technological domains.
Starting with transparency, it’s fascinating to think about how certain materials can allow light to pass through them almost unhindered. This isn’t just about being clear; it’s about the ability of the material to transmit light without absorbing it significantly. The unique internal structure of optical crystals is the key here.
At a molecular level, these crystals are arranged in such a way that they don’t absorb certain wavelengths of light. This is why some optical crystals can be perfectly transparent for visible light but might be opaque for ultraviolet or infrared light. The selective transparency of optical crystals is not just a passive property; it’s actively harnessed in various applications, from simple lens crafting to advanced laser technologies.
Next, we delve into the refractive index, a property that often goes hand in hand with transparency but is distinct in its implications. In simple terms, the refractive index of a material determines how much it can bend or refract light. Imagine a beam of light entering a crystal; as it moves from air (or another medium) into the crystal, it bends.
This bending is not arbitrary; it’s determined by the refractive index of the crystal. Different optical crystals have different refractive indices, and this variation is not just a matter of academic interest. It has profound implications in the world of optics. Lenses, for instance, rely on the refractive properties of materials to focus light. The precise bending of light in optical instruments, from microscopes to telescopes, is all down to the refractive index of the materials used.
Lastly, birefringence is a property that might sound complex but is fundamentally about the dual nature of light when it interacts with certain crystals. Some optical crystals don’t just have a single refractive index; they have two (or more) depending on the direction of light. This means that when light enters such a crystal, it can split into two distinct rays, each refracted differently.
This phenomenon can lead to fascinating optical effects. For instance, under certain conditions, a single point of light entering a birefringent crystal can emerge as two distinct points on the other side. This property is not just a curious optical trick; it’s used in various applications, especially in the study of material stresses and in certain types of optical filters.
In conclusion, the world of optical crystals is vast and intricate. The properties of transparency, refractive index, and birefringence are just the tip of the iceberg, but they offer a glimpse into the profound ways in which these materials interact with light. As science and technology advance, our understanding of these properties and our ability to harness them for new applications will only grow, opening up new frontiers in the realm of optics and photonics.
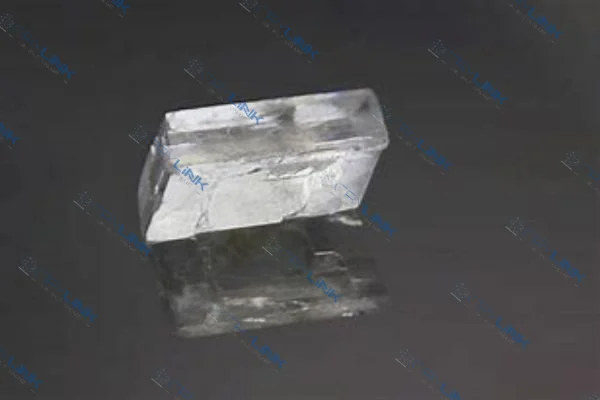
Lattice Structures and Their Influence on Optical Properties
The intricate world of optical crystals is deeply intertwined with their lattice structures. These structures, which refer to the repeating patterns of atoms or molecules within the crystal, play a pivotal role in determining the crystal’s optical properties.
The cubic lattice, known for its simplicity and symmetry, is a prime example. Found in crystals like diamond, this structure is characterized by its isotropic optical properties. In layman’s terms, this means that the way these crystals interact with light remains consistent, regardless of the direction from which the light approaches or travels through the crystal. This isotropy is particularly beneficial in applications where uniform optical behavior is essential, such as in certain types of lenses or optical instruments.
On the other hand, the tetragonal and orthorhombic lattices, with their reduced symmetry, introduce a fascinating phenomenon called birefringence. Crystals like calcite, which possess these lattice structures, have the unique ability to refract light differently along different axes. This results in the splitting of a light ray into two distinct paths when it passes through the crystal. Such behavior can be harnessed in various optical applications, including the development of specialized filters and imaging techniques.
Hexagonal and trigonal lattices, as seen in crystals like quartz, bring another layer of complexity to the table. These structures can induce optical activity in the crystal, allowing it to rotate the plane of polarization of light. This rotation can be exploited in numerous ways, especially in the field of optical communication and in devices that rely on the specific polarization of light.
However, it’s not just the intended lattice structures that influence optical properties. Sometimes, imperfections or defects in the lattice can have significant effects. These defects can create localized states within the crystal that have the ability to absorb certain wavelengths of light. As a result, some optical crystals may exhibit coloration, even if they are primarily transparent. This coloration, while sometimes unintended, can be used to identify, study, or even exploit specific properties of the crystal.
In essence, the lattice structures of optical crystals, whether perfect or flawed, are at the heart of their optical behaviors. Understanding these structures and their implications is crucial for anyone looking to harness the full potential of these remarkable materials.
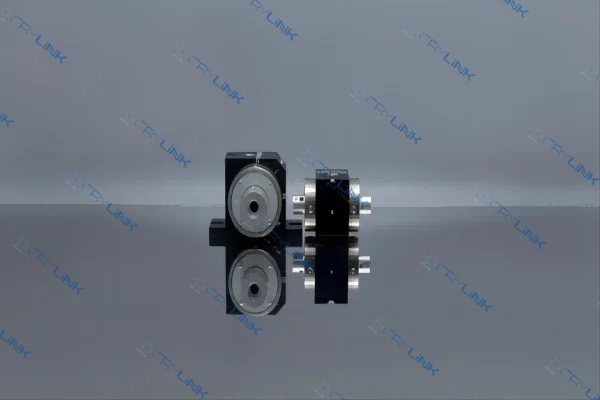
Applications of Optical Crystals
Optical crystals, with their unique properties and capabilities, have become indispensable in modern technology. Their ability to manipulate, amplify, and transmit light in specific ways has paved the path for numerous innovations and advancements.
One of the most prominent applications of optical crystals is in laser systems. Lasers, which stand for Light Amplification by Stimulated Emission of Radiation, rely heavily on the properties of these crystals.
By using crystals with specific lattice structures and impurities, scientists can produce lasers of different wavelengths, powers, and properties. For instance, ruby crystals are used to produce red lasers, while neodymium-doped yttrium aluminum garnet (Nd:YAG) crystals are used for infrared lasers. These lasers are then employed in a range of applications, from medical surgeries and tattoo removal to cutting metals and optical data storage.
Telecommunications is another domain where optical crystals play a pivotal role. The modern world’s data-driven needs require fast and efficient communication systems. Fiber-optic cables, which use light to transmit data over long distances with minimal loss, leverage the properties of optical crystals. These crystals are used in repeaters and amplifiers to boost the signal in long-distance transmissions, ensuring that data reaches its destination without degradation.
In the realm of imaging and microscopy, optical crystals have revolutionized the way we see the world, both at the macro and micro levels. High-quality lenses made from these crystals provide clearer and more detailed images. In advanced microscopes, birefringent crystals help researchers visualize minute details by manipulating the polarization of light, revealing structures and details that would otherwise remain hidden.
Furthermore, the field of astronomy has benefited immensely from optical crystals. Telescopes equipped with specialized crystal lenses and mirrors allow astronomers to gaze deep into the cosmos, capturing images of distant galaxies, stars, and planets with unparalleled clarity.
In essence, the applications of optical crystals are vast and varied. As technology continues to advance, it’s evident that the role of these crystals will only become more integral, driving innovations and breakthroughs in numerous fields. Their ability to interact with light in specialized ways ensures that they remain at the forefront of scientific and technological progress.
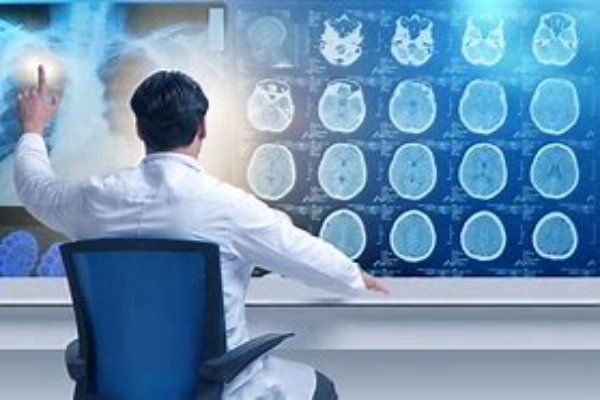
Future of Optical Crystals
With advancements in technology and deeper understanding, the potential applications and functionalities of optical crystals are bound to expand. Their role in future optical computing and quantum communication systems is particularly promising.
Conclusion
Optical crystals, with their unique properties and diverse applications, stand as a testament to the wonders of nature and the advancements of science. As we continue to explore and understand these materials, their potential to revolutionize the world of optics and beyond is undeniable.
FAQs
- What are the primary uses of optical crystals?
- Optical crystals are predominantly used in laser systems, telecommunications, imaging, and microscopy due to their unique light-manipulating properties.
- How does the lattice structure influence the optical properties of a crystal?
- The lattice structure determines the symmetry and arrangement of atoms in the crystal, which in turn influences properties like refractive index, birefringence, and optical activity.
- What is birefringence in optical crystals?
- Birefringence is a property where the crystal has different refractive indices along different axes, causing it to split a light ray into two distinct rays.
- Why are some optical crystals colored?
- The coloration in some optical crystals is due to defects in the lattice structure, which introduce localized states that can absorb specific wavelengths of light.
- Are all optical crystals naturally occurring?
- While many optical crystals are naturally occurring, some are synthetically produced in laboratories to achieve desired properties and purity levels.
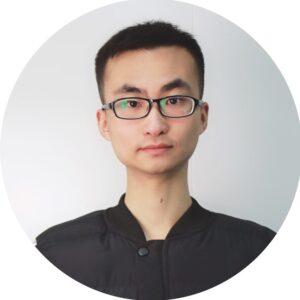
Frank
Frank graduated from the University of Shanghai for Science and Technology, majoring in optics. As a technical engineer at Crylink Company, he deeply understands crystal materials and laser components.
Related Video(s) with this Article
Related Product(s) with this Article
Related Application(s) with this Article