Chapter 1. Fundamentals of LBO Nonlinear Crystals
Crystal structure and physical properties of LBO nonlinear crystals:
LBO is a non-centrosymmetric crystal with a monoclinic structure, belonging to the space group C2 (point group 2). The crystal structure of LBO is composed of triborate (BO3) and lithium (Li) ions. The BO3 groups are arranged in a triangular configuration, forming a six-membered ring in the crystal structure. The Li ions occupy the interstitial sites between the BO3 groups.
LBO nonlinear crystals have excellent physical properties, such as high thermal stability, high damage threshold, and wide transparency range. The thermal conductivity of LBO is relatively low compared to other nonlinear crystals, which makes it more susceptible to thermal effects. However, this can be mitigated by using proper cooling techniques during crystal growth and device operation.
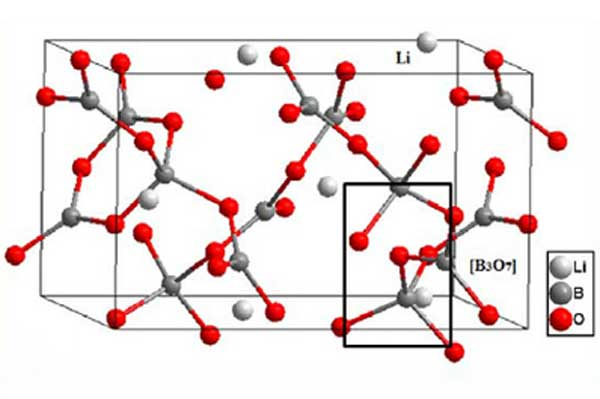
Phase-matching conditions in LBO nonlinear crystals:
One of the most critical properties of LBO nonlinear crystals is their ability to achieve phase-matching for efficient frequency conversion. Phase-matching is the condition where the wave vectors of the fundamental and harmonic waves match, enabling the conversion of the fundamental frequency into the harmonic frequency. The phase-matching condition in LBO crystals depends on several factors, such as the crystal orientation, temperature, and wavelength.
The most common method for achieving phase-matching in LBO nonlinear crystals is angle tuning, where the crystal is rotated to find the optimal angle that satisfies the phase-matching condition. The optimal angle depends on the type of nonlinear process, the wavelength, and the crystal thickness. Other methods for achieving phase-matching in LBO nonlinear crystals include temperature tuning and quasi-phase matching.
Nonlinear optical coefficients of LBO nonlinear crystals:
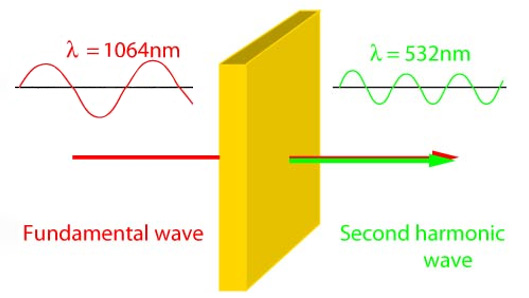
The nonlinear optical coefficients of LBO nonlinear crystals play a crucial role in determining the efficiency of frequency doubling and other nonlinear optical processes. The most important coefficient is the second-harmonic generation (SHG) coefficient, which is responsible for frequency doubling in LBO crystals. The SHG coefficient of LBO is approximately 1.5 times higher than that of potassium dihydrogen phosphate (KDP), a commonly used nonlinear crystal.
The SHG coefficient of LBO nonlinear crystals depends on several factors, such as crystal orientation, temperature, and crystal quality. The optimal crystal orientation for maximum SHG efficiency in LBO nonlinear crystals is the type I phase-matching direction, which corresponds to a 90-degree angle between the input and output polarizations.
Crystal growth methods for LBO nonlinear crystal production:
Several crystal growth methods have been developed for the production of high-quality LBO nonlinear crystals. The most commonly used methods are the Czochralski method, the flux method, and the top-seeded solution growth (TSSG) method.
The Czochralski method is a well-established technique for growing large, high-quality single crystals. It involves melting the starting materials in a crucible and slowly pulling a seed crystal from the melt while rotating the crystal to maintain uniform growth. The flux method is another common technique that involves dissolving the starting materials in a flux and slowly cooling the solution to form crystals.
The TSSG method is a relatively new technique that has gained popularity for its ability to produce high-quality crystals with low defect density. It involves using a seed crystal to initiate crystal growth from a supersaturated solution. The TSSG method can produce crystals with controlled defect density and orientation, which is essential for achieving optimal phase-matching conditions.
In conclusion, this chapter provided an overview of the fundamentals of LBO nonlinear crystals, including their crystal structure, physical properties, phase-matching conditions, nonlinear optical coefficients, and crystal growth methods. The understanding of these properties is crucial for designing and growing high-quality LBO nonlinear crystals for various applications
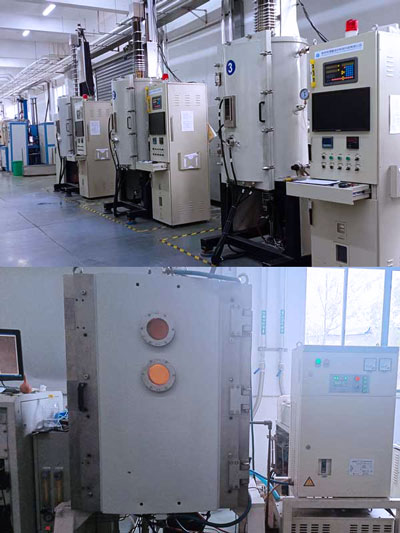
Chapter 2. Crystal Growth Techniques for LBO Nonlinear Crystals
Crystal growth is a crucial step in the production of high-quality LBO nonlinear crystals. The choice of crystal growth technique can significantly impact the crystal quality, defect density, and optical properties of the resulting crystal. This chapter discusses in detail the different crystal growth techniques used for the production of LBO nonlinear crystals.
Czochralski Method:
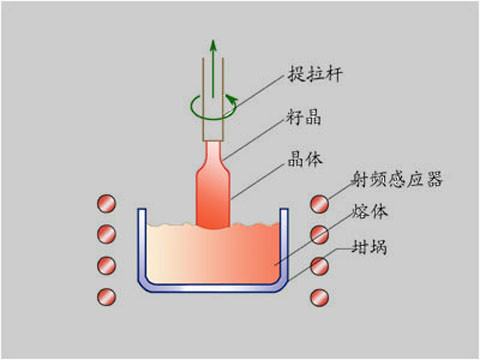
The Czochralski method is a well-established crystal growth technique that has been used for several decades in the production of high-quality single crystals. In this method, the starting materials, typically lithium oxide (Li2O) and boric oxide (B2O3), are melted in a crucible at high temperatures (above 900 °C). A seed crystal, typically a small LBO crystal, is slowly dipped into the melt and pulled up at a controlled rate while being rotated to maintain uniform growth. As the seed crystal is pulled up, it continuously melts and solidifies to form a single crystal.
The Czochralski method allows for the production of large LBO crystals with excellent crystal quality and low defect density. However, the method is relatively slow and requires careful control of the temperature, rotation, and pulling rate to maintain uniform growth. In addition, the high-temperature melt can react with the crucible material, leading to impurities and defects in the resulting crystal.
Flux Method:
The flux method is another commonly used technique for the production of LBO nonlinear crystals. In this method, the starting materials are dissolved in a flux, typically a mixture of potassium fluoride (KF) and boron oxide (B2O3), at high temperatures (above 800 °C). The solution is then slowly cooled to form LBO crystals.
The flux method allows for the production of large LBO crystals with low defect density and high optical quality. The use of a flux can help to reduce impurities and defects in the crystal, leading to improved crystal quality. However, the flux method can be challenging to control and can result in non-uniform crystal growth and irregular crystal shapes.
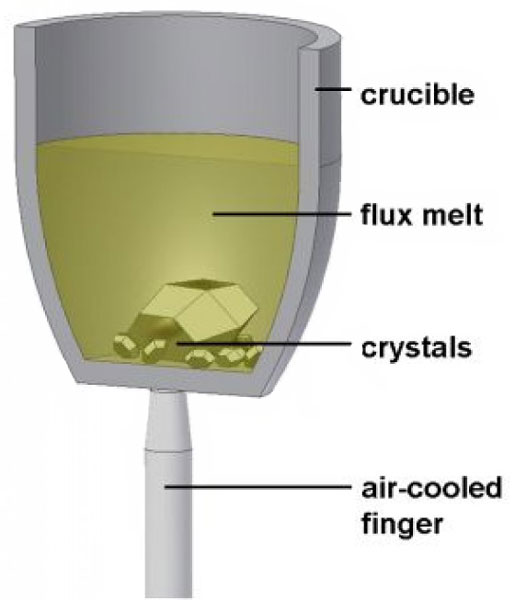
Top-Seeded Solution Growth (TSSG) Method:
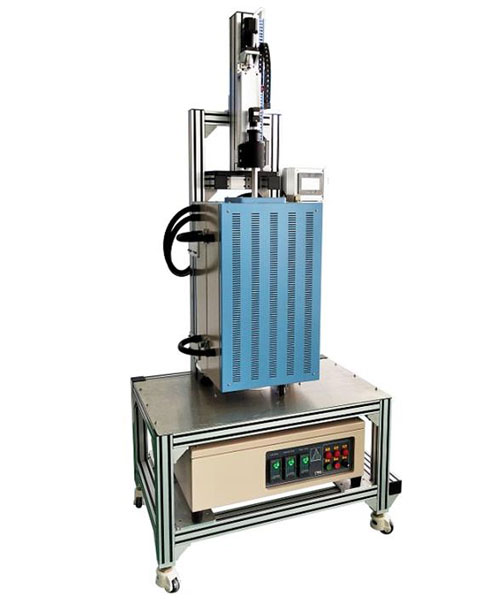
The top-seeded solution growth (TSSG) method is a relatively new technique that has gained popularity in recent years for the production of high-quality LBO nonlinear crystals. In this method, a small seed crystal is placed on top of a supersaturated solution of the starting materials, typically Li2O and B2O3, in a growth vessel. The solution is then slowly cooled to allow for crystal growth from the seed crystal.
The TSSG method allows for the production of high-quality LBO crystals with low defect density and excellent optical properties. The use of a seed crystal helps to control the crystal orientation and defect density, leading to improved crystal quality. The method is also relatively fast and can produce crystals with high growth rates. However, the TSSG method requires careful control of the cooling rate, seed crystal size, and concentration of the starting materials to maintain uniform crystal growth.
Hybrid Method:
A hybrid method that combines the advantages of the Czochralski and TSSG methods has also been developed for the production of LBO nonlinear crystals. In this method, a small seed crystal is first grown using the TSSG method, and then the crystal is attached to a rod and used as a seed for the Czochralski method. The resulting crystal is a hybrid crystal with a low defect density and high optical quality.
Conclusion:
Crystal growth is a critical step in the production of high-quality LBO nonlinear crystals. The choice of crystal growth technique can significantly impact the crystal quality and optical properties of the resulting crystal. The Czochralski, flux, and TSSG methods are the most commonly used techniques for the production of LBO nonlinear crystals, each with its advantages and disadvantages. The Czochralski method allows for the production of large single crystals with excellent quality, while the flux method can reduce impurities and defects in the crystal. The TSSG method provides precise control over crystal orientation and defect density, and the hybrid method combines the advantages of the TSSG and Czochralski methods.
The choice of crystal growth method depends on several factors, such as the desired crystal size, quality, and application requirements. In addition, the crystal growth parameters, such as temperature, cooling rate, and seed crystal size, must be carefully controlled to maintain uniform growth and achieve the desired crystal quality.
Overall, the crystal growth techniques discussed in this chapter provide valuable insights into the methods used for the production of high-quality LBO nonlinear crystals. The understanding of these techniques is crucial for the design and optimization of crystal growth processes and the production of high-quality LBO nonlinear crystals for various applications in optics and photonics.
Chapter 3. Characterization Methods for LBO Nonlinear Crystals
The characterization of LBO nonlinear crystals is essential for understanding their properties and optimizing their performance in various applications. This chapter discusses the different characterization methods used for LBO nonlinear crystals.
X-ray Diffraction (XRD):
X-ray diffraction is a powerful technique for determining the crystal structure and orientation of LBO nonlinear crystals. XRD involves shining a beam of X-rays onto the crystal and measuring the intensity and direction of the scattered X-rays. By analyzing the scattered X-rays, the crystal structure and orientation can be determined.
XRD can be used to identify the crystal structure and orientation of LBO nonlinear crystals, which is essential for achieving optimal phase-matching conditions in various applications. XRD can also be used to determine the crystal quality and defects in the crystal, such as twinning and dislocations.
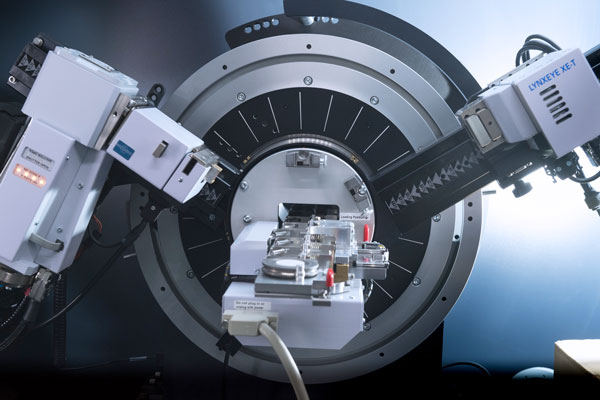
Optical Microscopy:
Optical microscopy is a commonly used technique for characterizing the surface morphology and defects in LBO nonlinear crystals. Optical microscopy involves illuminating the crystal with a beam of light and observing the reflected or transmitted light through a microscope. By analyzing the surface morphology and defects, the crystal quality can be determined.
Optical microscopy can be used to identify surface defects, such as scratches, cracks, and pits, that can affect the performance of the crystal in various applications. Optical microscopy can also be used to determine the crystal thickness and uniformity.
Raman Spectroscopy:
Raman spectroscopy is a powerful technique for characterizing the vibrational properties of LBO nonlinear crystals. Raman spectroscopy involves shining a laser beam onto the crystal and measuring the scattered light at different wavelengths. By analyzing the scattered light, the vibrational modes and frequencies of the crystal can be determined.
Raman spectroscopy can be used to identify impurities and defects in LBO nonlinear crystals that can affect their performance in various applications. Raman spectroscopy can also be used to determine the crystal quality and phase purity.
Second-Harmonic Generation (SHG) Measurement:
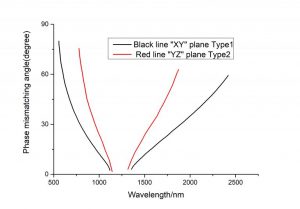
SHG measurement is a commonly used technique for characterizing the nonlinear optical properties of LBO nonlinear crystals. SHG measurement involves shining a laser beam of a specific wavelength onto the crystal and measuring the intensity of the second-harmonic wave generated by the crystal. By analyzing the intensity of the second-harmonic wave, the nonlinear optical coefficients of the crystal can be determined.
SHG measurement can be used to determine the nonlinear optical coefficients of LBO nonlinear crystals, which is essential for optimizing their performance in various applications. SHG measurement can also be used to determine the crystal quality and defects, such as domain structure and non-uniformity.
Photoluminescence (PL) Spectroscopy:
PL spectroscopy is a powerful technique for characterizing the luminescent properties of LBO nonlinear crystals. PL spectroscopy involves shining a laser beam onto the crystal and measuring the emitted light at different wavelengths. By analyzing the emitted light, the luminescent properties and defects of the crystal can be determined.
PL spectroscopy can be used to identify impurities and defects in LBO nonlinear crystals that can affect their performance in various applications. PL spectroscopy can also be used to determine the crystal quality and homogeneity.
Conclusion:
Characterization methods are essential for understanding the properties and optimizing the performance of LBO nonlinear crystals in various applications. XRD, optical microscopy, Raman spectroscopy, SHG measurement, and PL spectroscopy are the most commonly used techniques for characterizing LBO nonlinear crystals.
The choice of characterization method depends on several factors, such as the desired information, crystal size, and application requirements. The characterization parameters, such as laser wavelength, measurement conditions, and sample preparation, must be carefully controlled to obtain accurate and reliable results.
Overall, the characterization methods discussed in this chapter provide valuable insights into the methods used for the characterization of LBO nonlinear crystals. The understanding of these methods is crucial for the design and optimization of LBO nonlinear crystals for various applications in optics and photonics.
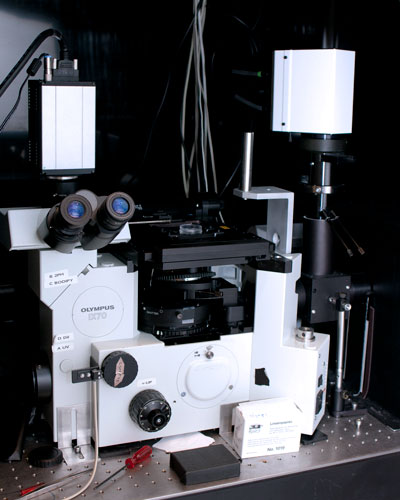
In addition to the methods discussed in this chapter, there are several other techniques that can be used for the characterization of LBO nonlinear crystals, such as atomic force microscopy (AFM), transmission electron microscopy (TEM), and Fourier transform infrared spectroscopy (FTIR). The choice of technique depends on the specific properties that need to be characterized, such as crystal structure, defects, and optical properties.
It is worth noting that the characterization methods discussed in this chapter are not limited to LBO nonlinear crystals and can be applied to other nonlinear crystals as well. The understanding and application of these methods are crucial for the development and optimization of various nonlinear optical devices and applications.
Chapter 4. Applications of LBO Nonlinear Crystals
LBO nonlinear crystals have unique properties that make them ideal for various applications in optics and photonics. This chapter discusses some of the most common applications of LBO nonlinear crystals.
Frequency Doubling:
One of the most common applications of LBO nonlinear crystals is frequency doubling, where the fundamental frequency of a laser beam is doubled to produce a higher frequency output. LBO nonlinear crystals have a high second-harmonic generation (SHG) coefficient, which makes them ideal for efficient frequency doubling.
Frequency doubling can be used in various applications, such as laser printing, laser cooling, and quantum optics. In laser printing, frequency doubling is used to produce high-quality images with sharp contrast and vibrant colors. In laser cooling, frequency doubling is used to cool atoms and molecules to extremely low temperatures for various applications, such as quantum computing and atomic clocks. In quantum optics, frequency doubling is used to generate entangled photon pairs for quantum information processing.
Optical Parametric Amplification (OPA):
Optical parametric amplification (OPA) is a nonlinear process that involves the conversion of a high-energy photon into two lower-energy photons. LBO nonlinear crystals have a high nonlinear coefficient, which makes them ideal for efficient OPA.
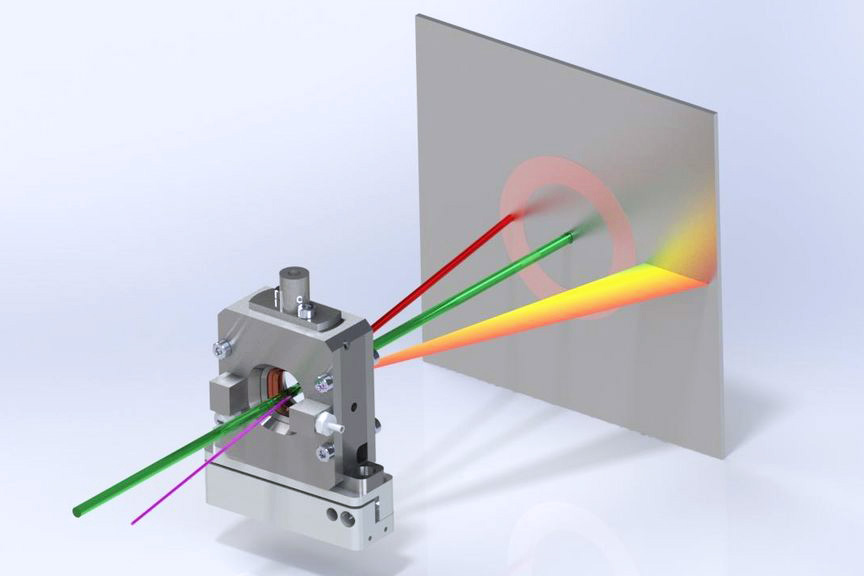
OPA can be used in various applications, such as biomedical imaging, spectroscopy, and telecommunications. In biomedical imaging, OPA is used to produce high-resolution images of biological tissues with improved contrast and reduced phototoxicity. In spectroscopy, OPA is used to generate tunable light sources for various applications, such as chemical analysis and material characterization. In telecommunications, OPA is used to amplify and convert signals in optical communication systems.
Sum-Frequency Generation (SFG):
Sum-frequency generation (SFG) is a nonlinear process that involves the conversion of two input photons into a single output photon with a frequency equal to the sum of the input frequencies. LBO nonlinear crystals have a high nonlinear coefficient, which makes them ideal for efficient SFG.
SFG can be used in various applications, such as surface science, molecular spectroscopy, and environmental monitoring. In surface science, SFG is used to study the surface properties of materials, such as the orientation and packing density of molecules on a surface. In molecular spectroscopy, SFG is used to probe the vibrational properties of molecules in various environments, such as liquids and gases. In environmental monitoring, SFG is used to detect pollutants and contaminants in water and air.
Optical Parametric Oscillation (OPO):
Optical parametric oscillation (OPO) is a nonlinear process that involves the conversion of a high-energy photon into two lower-energy photons in a resonant cavity. LBO nonlinear crystals have a high nonlinear coefficient, which makes them ideal for efficient OPO.
OPO can be used in various applications, such as quantum optics, spectroscopy, and metrology. In quantum optics, OPO is used to generate squeezed states of light for various applications, such as quantum communication and quantum sensing. In spectroscopy, OPO is used to generate tunable light sources for various applications, such as chemical analysis and material characterization. In metrology, OPO is used to generate frequency combs for precise frequency measurements.
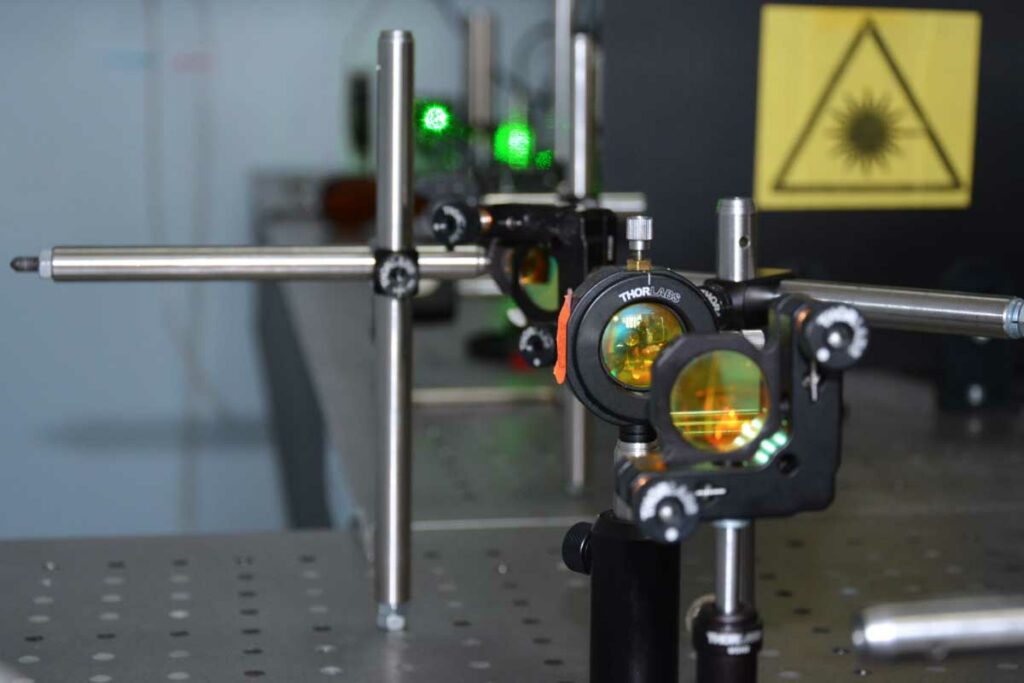
Conclusion:
LBO nonlinear crystals have unique properties that make them ideal for various applications in optics and photonics, such as frequency doubling, OPA, SFG, and OPO. The understanding and optimization of LBO nonlinear crystals are crucial for the development and optimization of various nonlinear optical devices and applications.
The applications discussed in this chapter are not limited to LBO nonlinear crystals and can be applied to other nonlinear crystals as well. The development and optimization of various nonlinear optical devices and applications rely on the understanding and application of these techniques.
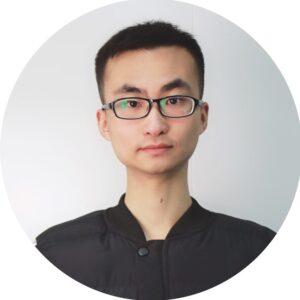
Frank
Frank graduated from the University of Shanghai for Science and Technology, majoring in optics. As a technical engineer at Crylink Company, he deeply understands crystal materials and laser components.
Related Video(s) with this Article
Related Product(s) with this Article
Related Application(s) with this Article