The Mastering BBO SHG Efficiency: Tips and Tricks for Optimal Performance (1) discusses Beta-barium borate (BBO) and its application in second harmonic generation (SHG) experiments. BBO, a nonlinear optical crystal, is used due to its high nonlinear coefficient, wide transparency range, and excellent optical homogeneity. It covers the fundamentals, advantages, limitations, and applications of BBO SHG, such as microscopy, spectroscopy, and laser systems. The article also discusses the process of BBO crystal growth and the issues that could arise during this process.
The experimental setup for BBO SHG, including laser source, optics, and beam shaping, are explained. Finally, it presents advanced techniques to enhance SHG efficiency and reduce noise, such as quasi-phase matching, temperature tuning, and cavity-enhanced SHG. This article continues with BBO SHG.
5. Applications of BBO SHG
BBO SHG is a versatile technique with many applications in various fields, including materials science, biology, medicine, and telecommunications. In this chapter, we will cover some of the most common applications of BBO SHG and their potential impact in different fields.
Materials Science
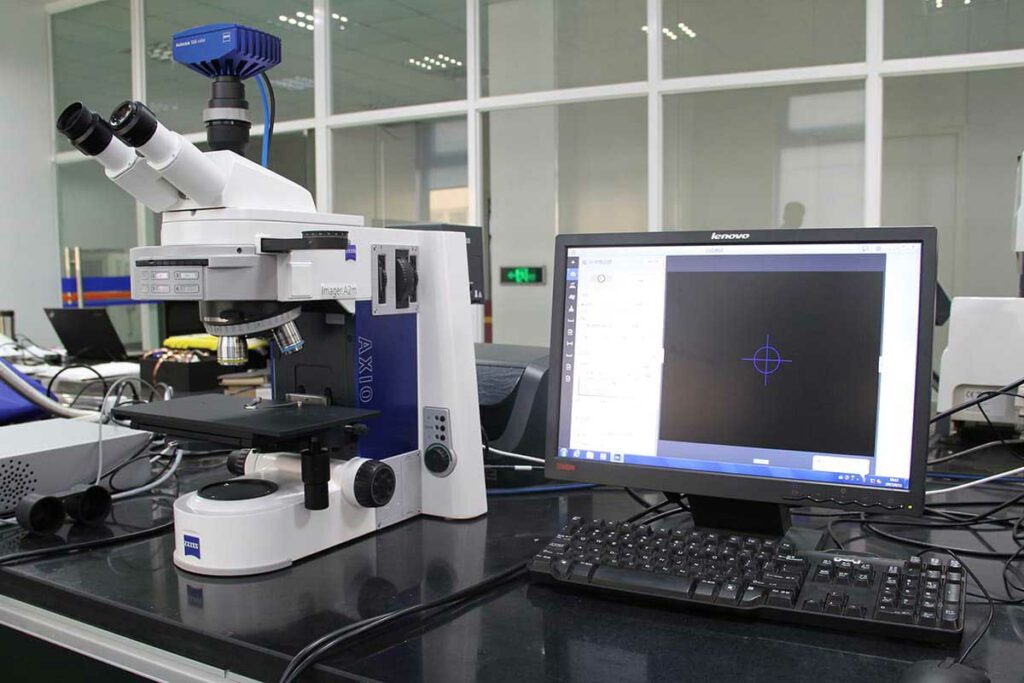
BBO SHG is a powerful tool for studying the properties of materials, including their crystal structure, morphology, and orientation. By using BBO SHG, researchers can investigate the nonlinear optical properties of materials and obtain high-resolution images of surfaces and interfaces. Barium metaborate SHG is commonly used in surface science, where it is used to investigate the electronic and structural properties of materials.
Biology and Medicine
BBO SHG is also a valuable tool in biology and medicine. Barium metaborate SHG can be used to study biological tissues’ structural and functional properties, including collagen, muscle fibers, and cell membranes. By using barium metaborate SHG, researchers can obtain high-resolution images of biological tissues without the need for staining or labeling. BBO SHG has applications in tissue engineering, cancer research, and neurobiology.
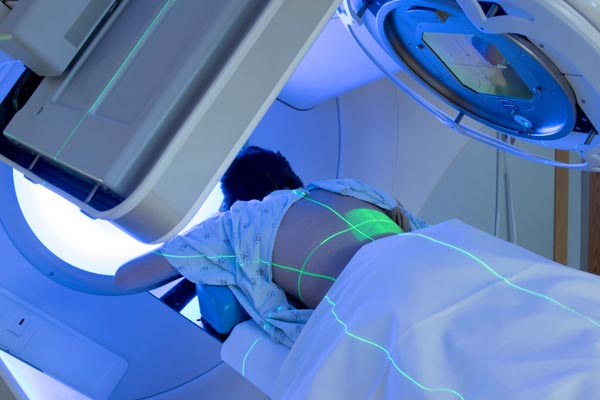
Telecommunications
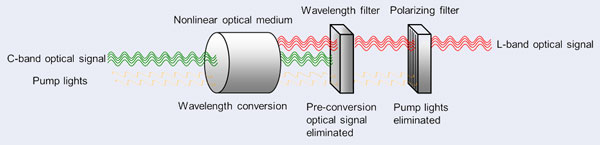
BBO SHG is also used in telecommunications for wavelength conversion and signal processing. By using barium metaborate SHG, researchers can convert optical signals from one wavelength to another, allowing for the transmission of information over long distances. Barium metaborate SHG is also used for optical switching and frequency doubling, which are crucial for optical communication systems.
Other Applications
BBO SHG has applications in various other fields, including environmental science, energy, and photonics. BBO SHG is used in environmental science for remote sensing and monitoring of atmospheric pollutants. BBO SHG is also used in energy research for studying energy conversion and storage materials. In photonics, BBO SHG is used for generating ultrashort laser pulses, which have applications in spectroscopy, microscopy, and laser micromachining.
Conclusion
Barium metaborate SHG is a versatile technique with many applications in various fields, including materials science, biology, medicine, and telecommunications. In this chapter, we have covered some of the most common applications of BBO SHG and their potential impact in different fields. Barium metaborate SHG has revolutionized the way researchers investigate the properties of materials, biological tissues, and optical communication systems. By understanding the diverse applications of barium metaborate SHG, researchers can continue to develop new and innovative uses for this powerful technique.
6. Limitations and Future Directions of BBO SHG
Barium metaborate SHG is a powerful technique with many applications in various fields. However, like all experimental techniques, BBO SHG has limitations that must be considered when designing experiments and interpreting results. In this chapter, we will cover the limitations of barium metaborate SHG and the future directions of this technique.
Limitations of BBO SHG
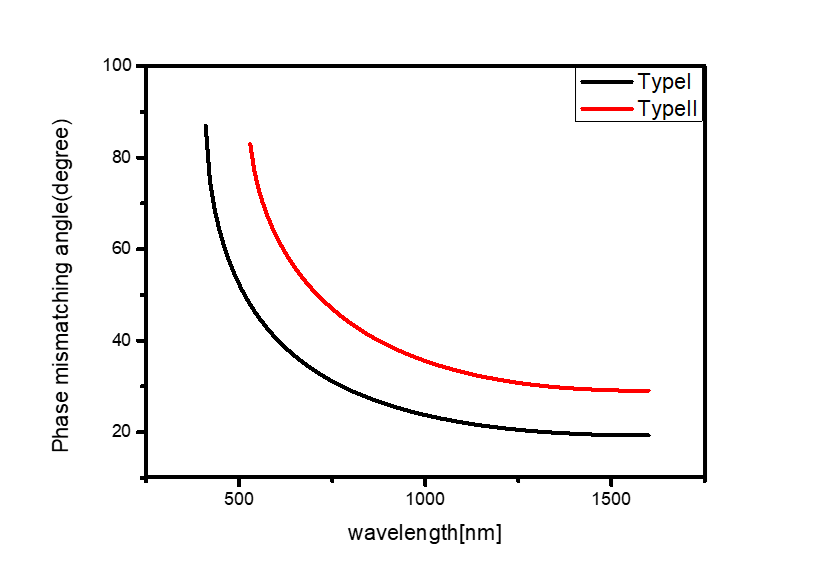
One of the primary limitations of barium metaborate SHG is its sensitivity to temperature and humidity. Barium metaborate crystals are hygroscopic, meaning they can absorb moisture from the environment, leading to changes in their optical properties. Temperature variations can also affect the crystal’s nonlinear coefficient and phase matching conditions, leading to changes in the SHG efficiency.
Another limitation of barium metaborate SHG is its susceptibility to damage from high laser powers. BBO crystals can be damaged by high laser intensities, leading to reduced SHG efficiency and even crystal fracture. Careful laser power control and crystal cooling must be implemented to avoid damage to the crystal.
Future Directions of BBO SHG
BBO SHG is a well-established technique with many applications in various fields. However, there is still much room for improvement and development. One area of research is the development of new crystal materials with enhanced nonlinear coefficients and improved thermal stability.
Another area of research is the development of new techniques for enhancing SHG efficiency and reducing noise. The use of resonant cavities, phase-matching techniques, and advanced noise reduction techniques can significantly improve the efficiency and quality of BBO SHG experiments.
Finally, there is a growing interest in the integration of BBO SHG with other experimental techniques, such as spectroscopy, microscopy, and imaging. By combining barium metaborate SHG with other techniques, researchers can obtain complementary information on the properties of materials, biological tissues, and optical communication systems.
Conclusion
BBO SHG is a powerful technique with many applications in various fields. However, like all experimental techniques, BBO SHG has limitations that must be considered when designing experiments and interpreting results. Temperature and humidity sensitivity and susceptibility to laser damage are some of the primary limitations of barium metaborate SHG. However, there is still much room for improvement and development. The future of barium metaborate SHG lies in the development of new crystal materials, techniques for enhancing SHG efficiency and reducing noise, and the integration with other experimental techniques. By addressing these challenges and developing new techniques, barium metaborate SHG will continue to be a valuable tool in research and technology.
7. Comparison of BBO SHG with Other Nonlinear Optical Techniques
Barium metaborate SHG is just one of many nonlinear optical techniques used in research and technology. In this chapter, we will compare BBO SHG with other commonly used nonlinear optical techniques, including two-photon microscopy, harmonic generation microscopy, and sum frequency generation spectroscopy.
Two-Photon Microscopy
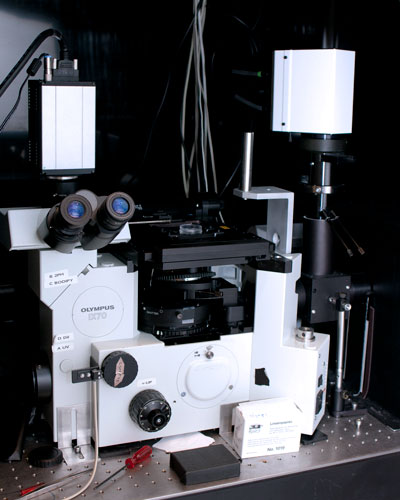
Two-photon microscopy is a powerful imaging technique that uses two-photon excitation to generate fluorescence in biological tissues. Two-photon microscopy is similar to BBO SHG in that it relies on nonlinear optical effects to generate signals. However, two-photon microscopy requires a femtosecond laser source and a specialized microscope setup, which can be more complicated than the setup required for barium metaborate SHG. Two-photon microscopy also has a lower spatial resolution than barium metaborate SHG, making it less suitable for imaging small structures such as collagen fibers.
Harmonic Generation Microscopy
Harmonic generation microscopy (HGM) is another imaging technique that uses nonlinear optical effects to generate signals. HGM relies on second harmonic generation, similar to BBO SHG, but can also use third and fourth harmonic generation. HGM has a higher spatial resolution than BBO SHG and can be used for imaging both biological tissues and materials. However, HGM requires specialized microscope setups and is less suitable for real-time imaging than BBO SHG.
Sum Frequency Generation Spectroscopy
Sum frequency generation spectroscopy (SFG) is a technique for studying the surface properties of materials, including their composition, orientation, and interactions. SFG relies on the sum frequency generation of two input beams, similar to barium metaborate SHG. However, SFG requires high-power laser sources and a sophisticated experimental setup, making it more challenging to implement than BBO SHG. SFG also has a lower spatial resolution than BBO SHG, making it less suitable for imaging small structures.
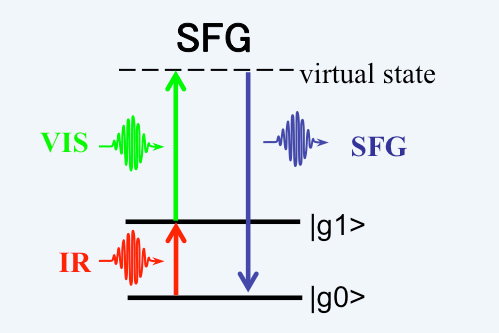
Comparison with BBO SHG
Barium metaborate SHG, two-photon microscopy, harmonic generation microscopy, and sum frequency generation spectroscopy are all powerful nonlinear optical techniques with many applications in various fields. However, each technique has its advantages and limitations.
Compared to two-photon microscopy, BBO SHG is simpler to implement and has a higher spatial resolution, making it better suited for imaging small structures. However, two-photon microscopy can generate fluorescence signals, making it more versatile for imaging biological tissues.
Compared to harmonic generation microscopy, BBO SHG is simpler to implement and has a lower photodamage risk. However, harmonic generation microscopy has a higher spatial resolution and can be used for imaging both biological tissues and materials.
Compared to sum frequency generation spectroscopy, barium metaborate SHG is simpler to implement and has a higher spatial resolution. However, sum frequency generation spectroscopy is better suited for studying surface properties of materials.
Conclusion
BBO SHG is just one of many nonlinear optical techniques used in research and technology. Two-photon microscopy, harmonic generation microscopy, and sum frequency generation spectroscopy are other powerful techniques with different advantages and limitations compared to BBO SHG. By understanding the differences between these techniques, researchers can choose the appropriate technique for their research and technology applications.
8. Tips and Tricks for Successful BBO SHG Experiments
In this final chapter, we will provide tips and tricks for conducting successful BBO SHG experiments. By following these guidelines, researchers can optimize their experimental setup and obtain high-quality results.
Choose the appropriate crystal size and orientation: The choice of crystal size and orientation can significantly impact SHG efficiency. Large crystals and specific crystal orientations can result in higher SHG efficiency.
Use a stable and high-quality laser source: The laser source used in barium metaborate SHG experiments should be stable and have a high beam quality. This ensures that the laser intensity and wavelength are consistent, leading to consistent SHG efficiency.
Use a temperature-controlled chamber or Peltier cooler: Temperature variations can affect the crystal’s nonlinear coefficient and phase matching conditions, leading to changes in SHG efficiency. Using a temperature-controlled chamber or Peltier cooler can ensure stable and consistent crystal temperature.
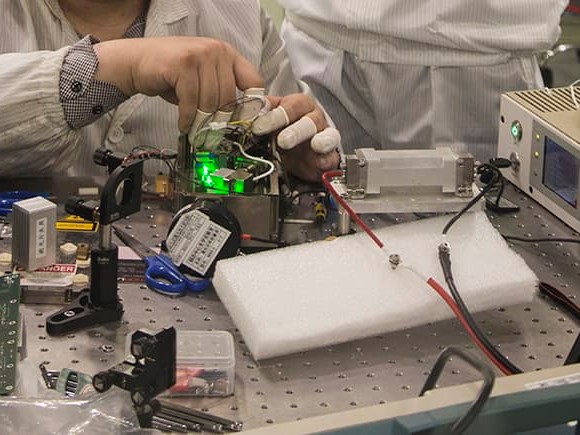
Control laser power: High laser powers can damage BBO crystals, leading to reduced SHG efficiency and even crystal fracture. Careful laser power control and crystal cooling must be implemented to avoid damage to the crystal.
Use balanced detectors for noise reduction: Common-mode noise can arise from various sources, including power fluctuations and laser intensity noise. By using balanced detectors, common-mode noise can be rejected, leading to higher signal-to-noise ratios.
Choose appropriate filters: Filters can be used to remove unwanted wavelengths and reduce noise in BBO SHG experiments. Choosing the appropriate filters can significantly improve SHG efficiency and reduce noise.
Monitor crystal performance: It is essential to monitor the crystal’s performance throughout the experiment to ensure stable and consistent SHG efficiency. Regularly measuring the SHG signal and crystal temperature can detect any changes in performance and allow for adjustments to be made.
Understand the limitations of barium metaborate SHG: As with all experimental techniques, BBO SHG has limitations that must be considered when designing experiments and interpreting results. Temperature and humidity sensitivity and susceptibility to laser damage are some of the primary limitations of BBO SHG.
Conclusion
Barium metaborate SHG is a powerful technique for studying the nonlinear optical properties of materials. By following these tips and tricks, researchers can optimize their experimental setup and obtain high-quality results. The appropriate crystal size and orientation, laser source, temperature control, laser power control, balanced detectors, filters, and crystal performance monitoring are all essential factors in conducting successful BBO SHG experiments. By understanding the limitations of barium metaborate SHG and following these guidelines, researchers can use this powerful technique to advance their research and technology applications.
Overall Conclusion:
In conclusion, BBO SHG is a powerful and versatile technique for studying the nonlinear optical properties of materials. It has many applications in various fields, including materials science, biology, medicine, and telecommunications. BBO SHG is used for imaging small structures and generating high-resolution images of surfaces and interfaces. It is also used for wavelength conversion, signal processing, and optical switching, which are crucial for optical communication systems.
While barium metaborate SHG has some limitations, such as sensitivity to temperature and humidity, and susceptibility to laser damage, careful experimental design and monitoring can help researchers overcome these limitations. In addition, ongoing research on new crystal materials, techniques for enhancing SHG efficiency and reducing noise, and integration with other experimental techniques will continue to improve the capabilities and applications of BBO SHG.
To conduct successful BBO SHG experiments, researchers should follow best practices such as choosing the appropriate crystal size and orientation, using a stable and high-quality laser source, controlling temperature and laser power, using balanced detectors and appropriate filters, and monitoring crystal performance.
In summary, BBO SHG is a valuable tool in research and technology applications. By understanding the fundamental principles of BBO SHG, its applications and limitations, and best practices for experimental design, researchers can continue to advance the field and use this powerful technique to address new and exciting research questions.
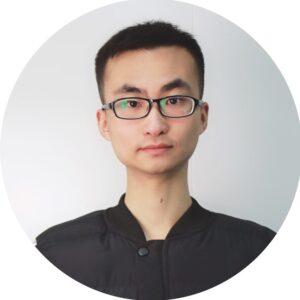
Frank
Frank graduated from the University of Shanghai for Science and Technology, majoring in optics. As a technical engineer at Crylink Company, he deeply understands crystal materials and laser components.
Related Video(s) with this Article
Related Product(s) with this Article
Related Application(s) with this Article