Chapter 1: Fundamentals of Laser Physics
This chapter provides an overview of the principles of laser physics, including the different types of lasers, laser gain media, laser cavity design, and laser modes. The chapter discusses the properties of Ti: sapphire crystals, such as their crystal structure, doping levels, and spectral characteristics, that make them suitable for laser applications. The chapter also covers the basics of laser pumping, including the different pumping mechanisms and the importance of choosing the right pump source.
The chapter also introduces the concept of mode-locking, a critical technique used in Ti: sapphire lasers to generate ultrafast pulses. The chapter explains the various mode-locking techniques, such as passive and active mode-locking, and the role of saturable absorbers in mode-locking. The chapter also discusses the importance of dispersion management in mode-locked lasers and how it affects pulse duration.
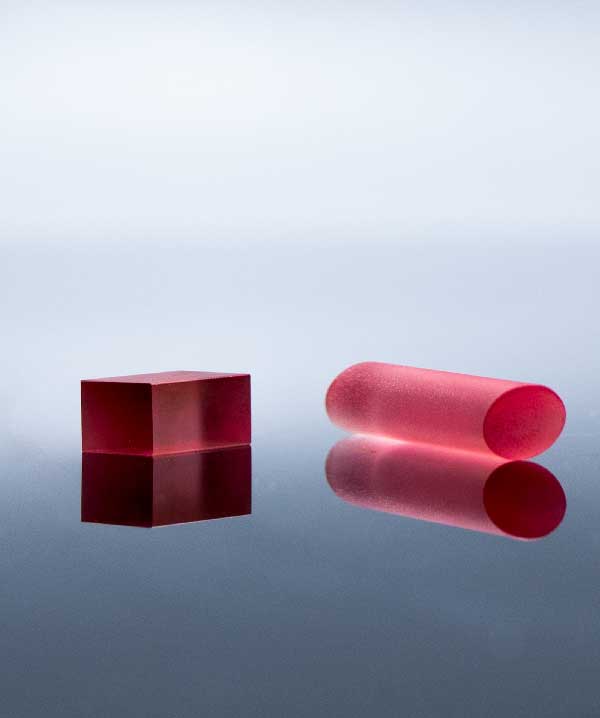
Laser Physics Fundamentals
Lasers produce coherent, monochromatic, and collimated light through stimulated emission. The stimulated emission process occurs in a laser gain medium, which can be a solid, liquid, or gas. The gain medium is excited by an external energy source, the pump source, which raises the electrons to an excited state. As the excited electrons relax to their ground state, they emit photons of the same energy and phase as the incident photons, resulting in coherent light.
Several types of lasers exist, such as solid-state, gas, and semiconductor. Each type of laser has unique properties, such as laser wavelength, output power, and tunability. Ti: sapphire lasers belong to the solid-state laser category and are known for their broad tunability, high power, and ultrafast pulse duration.
Laser Physics Fundamentals
Lasers produce coherent, monochromatic, and collimated light through stimulated emission. The stimulated emission process occurs in a laser gain medium, which can be a solid, liquid, or gas. The gain medium is excited by an external energy source, the pump source, which raises the electrons to an excited state. As the excited electrons relax to their ground state, they emit photons of the same energy and phase as the incident photons, resulting in coherent light.
Several types of lasers exist, such as solid-state, gas, and semiconductor. Each type of laser has unique properties, such as laser wavelength, output power, and tunability. Ti: sapphire lasers belong to the solid-state laser category and are known for their broad tunability, high power, and ultrafast pulse duration.
Ti: Sapphire Crystals Properties
Ti: sapphire crystals are made of titanium-doped sapphire and have unique properties that make them suitable for laser applications. The Ti: sapphire crystal structure consists of a hexagonal close-packed array of oxygen ions with titanium ions occupying the octahedral sites. The doping level of Ti ions in sapphire crystals can range from a few parts per million to a few percent, depending on the application.
Ti: sapphire crystals have a broad absorption spectrum in the visible and near-infrared region, making them suitable for a wide range of pump sources. The crystal’s broad emission spectrum, centered around 800 nm, makes them suitable for ultrafast laser applications. The crystal’s spectral characteristics can be tailored by adjusting the doping concentration and crystal length, allowing for fine-tuning of the laser output.
Laser Pumping
Ti: sapphire lasers can be pumped using various mechanisms, such as flashlamps, diodes, and fiber lasers. Flashlamp pumping is a common pumping mechanism used in Ti: sapphire lasers. A high-intensity flash of light pumps the Ti: sapphire crystal, which excites the electrons to the upper laser level. Diode-pumped Ti: sapphire lasers use semiconductor diode lasers as the pump source, providing a more efficient and compact pumping mechanism.
Choosing the right pump source is critical to achieving the desired laser performance. The pump source’s wavelength, pulse duration, and intensity can affect the laser output power, pulse duration, and stability. The choice of pump source also affects the cooling mechanism dissipating the heat generated during pumping.
Mode-Locking
Mode-locking is a technique used to generate ultrafast pulses in lasers. In Ti: sapphire lasers, mode-locking is achieved using saturable absorbers, which allow for the generation of ultrashort pulses with pulse durations in the femtosecond range. Passive mode-locking is achieved using a saturable absorber, such as a semiconductor saturable absorber mirror (SESAM). In contrast, active mode-locking is achieved using an external modulator, such as an acousto-optic modulator (AOM).
The pulse duration in a mode-locked laser is determined by the cavity’s round-trip time and the laser gain medium’s group velocity dispersion (GVD). The GVD measures the variation of the refractive index concerning the optical frequency. It affects the temporal broadening of the pulses as they travel through the laser cavity. Dispersion management techniques, such as prism and grating-based compensation, are used to compensate for the GVD and achieve ultrashort pulse durations.
Conclusion:
Chapter 1 provides an overview of the fundamentals of laser physics, the properties of Ti: sapphire crystals, and the importance of choosing the right pump source for Ti: sapphire lasers. The chapter also introduces the concept of mode-locking and its role in generating ultrafast pulses in Ti: sapphire lasers. Dispersion management is also discussed in the context of mode-locked lasers, and the various techniques used to compensate for GVD are covered. The chapter serves as a foundation for the subsequent chapters, which delve into the details of Ti: sapphire laser design, fabrication, and modification.
Chapter 2: Ti: Sapphire Laser Design
In this chapter, we discuss the design considerations for Ti: sapphire lasers, including the choice of pump source, the laser cavity design, and the choice of output coupling. The chapter covers the different types of pump sources, such as flashlamps and diode-pumped lasers, and their advantages and disadvantages. The chapter also discusses the various cavity designs, such as linear and ring cavities, and their impact on laser performance.
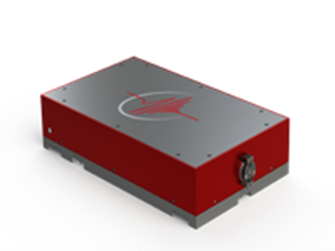
Pump Sources for Ti: Sapphire Lasers
Flashlamp pumping is a common pumping mechanism used in Ti: sapphire lasers, providing high peak power at a low cost. However, flashlamp pumping is inefficient, generating heat that needs to be dissipated from the laser crystal. Diode-pumped lasers provide a more efficient pumping mechanism, with higher pump power and longer pump lifetime. However, diode-pumped lasers are more expensive than flashlamp-pumped lasers, requiring more precise alignment.
Laser Cavity Design
The laser cavity is the optical resonator that contains the laser gain medium and the mirrors that define the laser mode. The laser cavity design affects the output power, pulse duration, and spectral bandwidth. Linear cavities are the simplest type of laser cavity and consist of two mirrors facing each other, with the laser gain medium between them. Ring cavities are more complex and consist of two curved mirrors and a folding mirror that creates a closed-loop path for the laser beam.
The choice of cavity design affects the laser output power, pulse duration, and spectral bandwidth. Ring cavities are typically more stable and produce higher output power than linear cavities. However, ring cavities require more precise alignment and are more complex to build and operate.
Output Coupling
The choice of output coupling affects the laser output power and pulse duration. Output coupling is the process of extracting a fraction of the laser power from the laser cavity. The output coupler is typically a partially reflecting mirror that reflects a fraction of the laser power and transmits the rest. The choice of output coupling affects the laser output power and pulse duration, with lower output coupling ratios producing higher output power and longer pulse durations.
Conclusion:
Chapter 2 provides an in-depth understanding of the design considerations for Ti: sapphire lasers. The chapter covers the different types of pump sources, their advantages and disadvantages, and the impact of the pump source on laser performance. The chapter also discusses the various cavity designs, their impact on laser performance, and the importance of choosing the right output coupling. The chapter provides a foundation for the subsequent chapters, which delve into the details of Ti: sapphire laser fabrication and modification.
Chapter 3: Ti: Sapphire Crystal Growth
This chapter discusses the process of growing Ti: sapphire crystals and the factors that affect crystal quality and performance. The chapter covers the different crystal growth techniques, such as the Czochralski and the heat exchanger methods, and their advantages and disadvantages. The chapter also discusses the different growth conditions, such as temperature, pressure, and crystal orientation, and their impact on crystal quality and performance.
Crystal Growth Techniques
The Czochralski method is a common crystal growth technique for Ti: sapphire crystals. In this method, a seed crystal is dipped into a molten sapphire and titanium oxide mixture. As the seed crystal is slowly withdrawn from the mixture, it pulls out of the melt. The crystal is then annealed to remove any defects or stress induced during growth.
The heat exchanger method is another crystal growth technique used for Ti: sapphire crystals. In this method, a sapphire rod is placed inside a quartz tube and heated by a radio frequency (RF) coil. The tube is filled with a sapphire and titanium oxide mixture, and the sapphire rod’s heat causes the mixture to melt and crystallize onto the rod.
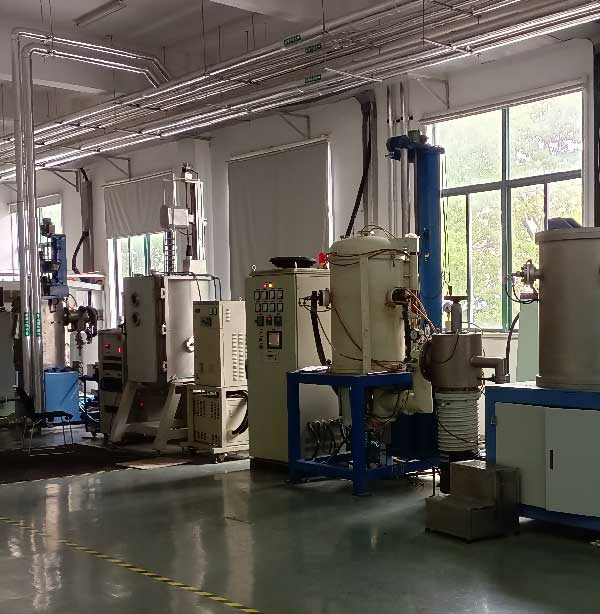
Growth Conditions
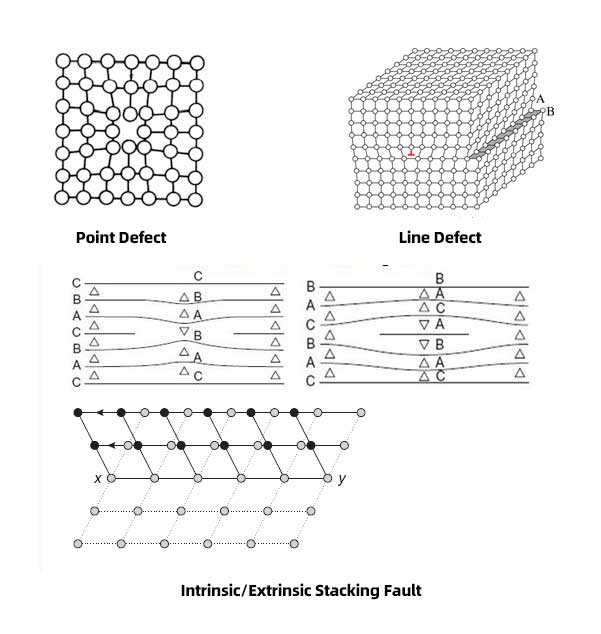
The growth conditions, such as temperature, pressure, and crystal orientation, significantly impact the crystal quality and performance. The crystal growth temperature affects the crystal’s growth rate, crystal structure, and crystal quality. The crystal growth pressure affects the crystal’s oxygen concentration and mechanical properties. The crystal orientation affects its spectral characteristics and ability to withstand high optical intensities.
Crystal quality is a critical factor in Ti: sapphire laser performance, as crystal defects and impurities, can affect the laser’s output power and pulse duration. Defects such as dislocations, cracks, and inclusions can act as scattering sites for the laser beam, reducing the laser’s efficiency and output power. Impurities such as iron and chromium can affect the crystal’s spectral characteristics and ability to produce ultrafast pulses.
Conclusion:
Chapter 3 provides an in-depth understanding of the crystal growth techniques used for Ti: sapphire crystals and the factors that affect crystal quality and performance. The chapter covers the different crystal growth techniques, their advantages and disadvantages, and the impact of growth conditions on crystal quality and performance. The chapter provides a foundation for the subsequent chapters, which delve into the details of Ti: sapphire laser fabrication and modification.
Chapter 4: Ti: Sapphire Laser Fabrication
In this chapter, we discuss the process of fabricating Ti: sapphire lasers, including the preparation of the laser gain medium, the assembly of the laser cavity, and the alignment of the laser components. The chapter covers the different methods used to mount and cool the laser gain medium, such as water-cooled and air-cooled mounts. The chapter also discusses the techniques used to align the laser components and the importance of proper alignment in achieving optimal laser performance.
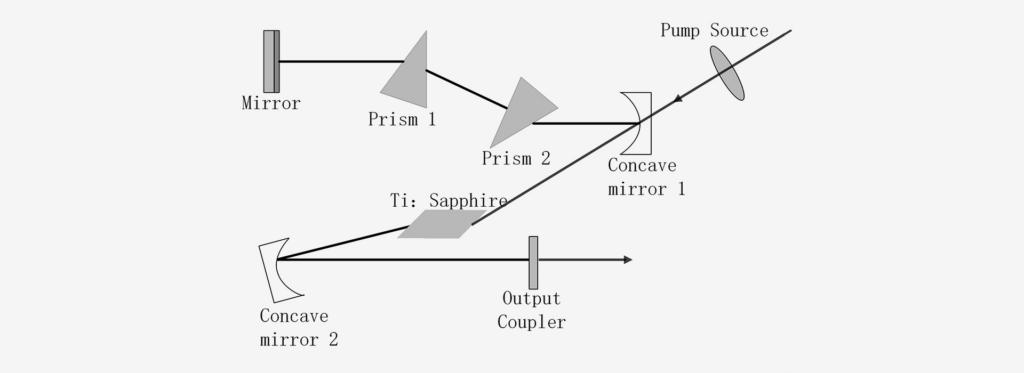
Laser Gain Medium Preparation
The laser gain medium is typically Ti: a sapphire crystal was grown using one of the crystal growth techniques discussed in Chapter 3. The crystal is prepared for laser operation by polishing the ends and coating them with dielectric or metal mirrors. The coating reflects the laser beam into the crystal, creating the optical resonator that produces the laser output.
Laser Cavity Assembly
The laser cavity consists of the laser gain medium and the mirrors that define the laser mode. The cavity design affects the laser output power, pulse duration, and spectral bandwidth. The cavity is assembled by mounting the laser gain medium in a suitable holder, such as a water-cooled or air-cooled mount, and aligning the mirrors to achieve the desired cavity geometry.
Laser Component Alignment
The alignment of the laser components is critical to achieving optimal laser performance. Misalignment of the laser components can cause optical losses, reduce the laser output power, and increase the pulse duration. The alignment of the laser components is typically performed using optical and mechanical alignment techniques, such as a He-Ne laser or a microscope. The alignment procedure involves adjusting the position and angle of the laser components to achieve optimal cavity geometry.
Cooling and Thermal Management
Cooling and thermal management are critical factors in Ti: sapphire laser operation, as excessive heat can degrade the laser performance and reduce the laser’s lifetime. The laser gain medium is typically mounted in a water-cooled or air-cooled mount to dissipate the heat generated during laser operation. The cooling system is designed to maintain the crystal temperature within a narrow range to ensure stable laser operation and optimal laser performance.
Conclusion:
Chapter 4 provides an in-depth understanding of the Ti: sapphire laser fabrication process, including the preparation of the laser gain medium, the assembly of the laser cavity, and the alignment of the laser components. The chapter covers the different methods used to mount and cool the laser gain medium, the importance of proper alignment, and the role of cooling and thermal management in achieving optimal laser performance. The chapter provides a foundation for the subsequent chapters, which delve into the details of Ti: sapphire laser modification and customization.
Chapter 5: Ti: Sapphire Laser Modification
In this chapter, we discuss the various modifications that can be made to Ti: sapphire lasers to tailor their output for specific applications. The chapter covers the techniques for modifying the laser output spectrum, pulse duration, and output power. The chapter also discusses the trade-offs associated with laser modification and the impact of modified laser parameters on laser performance.
Spectral Modification
The spectral characteristics of Ti: sapphire lasers can be modified using various techniques, such as wavelength tuning, frequency doubling, and optical parametric amplification (OPA). Wavelength tuning is achieved by adjusting the cavity length or the angle of the mirrors, while frequency doubling is achieved by using nonlinear crystals to double the laser frequency. OPA is a technique that allows for the generation of tunable wavelengths by exploiting the nonlinear properties of a nonlinear crystal.
Pulse Duration Modification
The pulse duration of Ti: sapphire lasers can be modified using various techniques, such as cavity dumping, pulse compression, and chirped pulse amplification (CPA). Cavity dumping is a technique that allows for the extraction of a single pulse from a mode-locked laser cavity by releasing the stored energy in the cavity. Pulse compression is a technique that reduces pulse duration by using dispersive elements such as prisms or gratings. CPA is a technique that allows for the amplification of chirped pulses by using a chirped pulse amplifier.
Output Power Modification
The output power of Ti: sapphire lasers can be modified using various techniques, such as Q-switching and gain switching. Q-switching is a technique that allows for extracting high-energy pulses from a mode-locked laser cavity by using an external modulator to increase the cavity loss. Gain switching is a technique that generates high-energy pulses by increasing the laser gain for a short period.
Trade-Offs and Impact on Laser Performance
The modifications made to Ti: sapphire lasers have trade-offs that affect laser performance. Spectral modification techniques, such as frequency doubling and OPA, can reduce the laser output power and increase the pulse duration. Pulse duration modification techniques, such as cavity dumping and pulse compression, can reduce the laser output power and increase the spectral bandwidth. Output power modification techniques, such as Q-switching and gain switching, can reduce the pulse duration and increase the spectral bandwidth.
Conclusion:
Chapter 5 provides an in-depth understanding of the various modifications that can be made to Ti: sapphire lasers to tailor their output for specific applications. The chapter covers the techniques for modifying the laser output spectrum, pulse duration, and output power. The chapter also discusses the trade-offs associated with laser modification and the impact of modified laser parameters on laser performance. The chapter provides a foundation for the subsequent chapters, which delve into Ti: sapphire laser customization and optimization.
Chapter 6: Ti: Sapphire Laser Customization
This chapter discusses the customization of Ti: sapphire lasers to meet specific application requirements. The chapter covers the different techniques for customizing the laser output, such as beam shaping, polarization control, and temporal synchronization. The chapter also discusses the importance of laser customization in achieving optimal laser performance and the impact of customized laser parameters on laser applications.
Beam Shaping
Beam shaping is a technique that modifies the laser beam profile to meet specific application requirements. Beam shaping techniques include apertures, lenses, and mirrors to modify the laser beam’s shape and size. The beam shape and size can be customized to optimize the laser’s interaction with the target material or to improve the laser’s efficiency.
Polarization Control
Polarization control is a technique used to modify the polarization state of the laser beam to meet specific application requirements. Polarization control techniques include using polarizers, waveplates, and birefringent crystals to modify the laser beam’s polarization state. The polarization state can be customized to optimize the laser’s interaction with the target material or to improve the laser’s efficiency.
Temporal Synchronization
Temporal synchronization is a technique that synchronizes the laser output with other devices, such as cameras or detectors, to meet specific application requirements. Temporal synchronization techniques include delay lines, acousto-optic modulators, and electro-optic modulators to control the laser output’s timing. The laser output’s timing can be customized to optimize the laser’s interaction with the target material or to improve the laser’s efficiency.
Impact on Laser Performance and Applications
The customization of Ti: sapphire lasers significantly impacts laser performance and applications. Customized laser parameters, such as beam shape, polarization state, and temporal synchronization, can significantly affect the laser’s interaction with the target material and efficiency. Customization can also improve the laser’s performance in specific applications, such as material processing, biomedical imaging, and spectroscopy.
Conclusion:
Chapter 6 provides an in-depth understanding of the customization of Ti: sapphire lasers to meet specific application requirements. The chapter covers the different techniques for customizing the laser output, such as beam shaping, polarization control, and temporal synchronization. The chapter also discusses the importance of laser customization in achieving optimal laser performance and the impact of customized laser parameters on laser applications. The chapter provides a foundation for the subsequent chapters, which delve into the details of Ti: sapphire laser optimization and applications.
Chapter 7: Ti: Sapphire Laser Optimization
In this chapter, we discuss the optimization of Ti: sapphire lasers for specific applications, including material processing, biomedical imaging, and spectroscopy. The chapter covers the techniques used to optimize the laser output, such as pulse shaping, pulse compression, and cavity optimization. The chapter also discusses the importance of laser optimization in achieving optimal laser performance and the impact of optimized laser parameters on laser applications.
Pulse Shaping
Pulse shaping is a technique used to modify the temporal profile of the laser pulses to optimize the laser’s interaction with the target material. Pulse shaping techniques include acousto-optic modulators, pulse stretchers, and pulse compressors to modify the laser pulse’s shape and duration. Pulse shaping can improve the laser’s efficiency in material processing and biomedical imaging applications.
Pulse Compression
Pulse compression is a technique that reduces the laser pulse duration to improve the laser’s interaction with the target material. Pulse compression techniques include chirped pulse amplification, dispersion compensation, and compression gratings to reduce the laser pulse duration. Pulse compression can improve the laser’s efficiency in material processing and spectroscopy applications.
Cavity Optimization
Cavity optimization is a technique to optimize the laser cavity’s geometry and components to improve laser performance. Cavity optimization techniques include using stable resonators, thermal management systems, and high-reflectivity mirrors to reduce cavity losses and improve laser efficiency. Cavity optimization can improve the laser’s output power and pulse duration, making it more suitable for specific applications.
Impact on Laser Performance and Applications
The optimization of Ti: sapphire lasers has a significant impact on laser performance and applications. Optimized laser parameters, such as pulse shape, pulse duration, and cavity geometry, can significantly affect the laser’s interaction with the target material and efficiency. Optimization can also improve the laser’s performance in specific applications, such as material processing, biomedical imaging, and spectroscopy.
Conclusion:
Chapter 7 provides an in-depth understanding of optimizing Ti: sapphire lasers for specific applications. The chapter covers the techniques used to optimize the laser output, such as pulse shaping, pulse compression, and cavity optimization. The chapter also discusses the importance of laser optimization in achieving optimal laser performance and the impact of optimized laser parameters on laser applications. The chapter provides a foundation for the subsequent chapters, which discuss the different applications of Ti: sapphire lasers in various fields.
Chapter 8: Applications of Ti: Sapphire Lasers
This chapter discusses the various applications of Ti: sapphire lasers in different fields, including material processing, biomedical imaging, and spectroscopy. The chapter covers the techniques used to tailor the laser output for specific applications and the advantages of using Ti: sapphire lasers in these fields.
Material Processing
Ti: sapphire lasers have numerous applications in material processing, such as micromachining, drilling, and welding. The laser’s high peak power and ultrafast pulse duration make it ideal for processing materials that are difficult to machine with conventional methods. Ti: sapphire lasers can also be customized to optimize the laser’s interaction with the target material, such as beam shaping and pulse shaping.
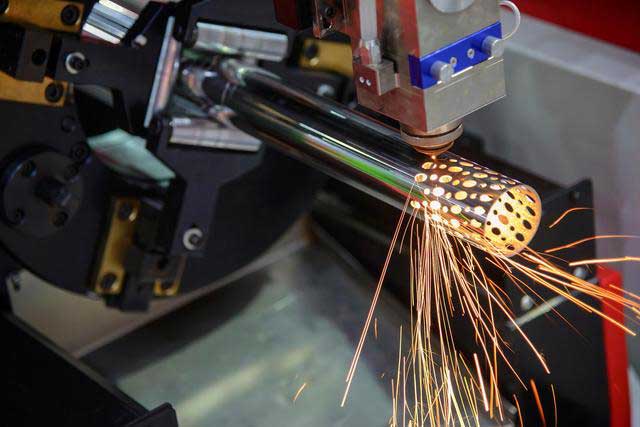
Biomedical Imaging
Ti: sapphire lasers have found widespread use in biomedical imaging applications, such as multiphoton microscopy, optical coherence tomography, and fluorescence lifetime imaging. The laser’s short pulse duration and high peak power allow for deep-tissue imaging with high resolution and minimal damage to the tissue. Ti: sapphire lasers can also be customized to optimize the laser’s interaction with the tissue, such as polarization control and temporal synchronization.
Spectroscopy
Ti: sapphire lasers have numerous applications in spectroscopy, such as time-resolved and ultrafast spectroscopy. The laser’s high repetition rate and pulse duration allow studying molecular dynamics and chemical reactions with high temporal resolution. Ti: sapphire lasers can also be customized to optimize the laser’s spectral characteristics, such as spectral broadening and wavelength tuning.
Conclusion:
Chapter 8 provides an in-depth understanding of the various applications of Ti: sapphire lasers in different fields, including material processing, biomedical imaging, and spectroscopy. The chapter covers the techniques used to tailor the laser output for specific applications and the advantages of using Ti: sapphire lasers in these fields. The chapter highlights the versatility of Ti: sapphire lasers and their ability to be customized for specific applications.
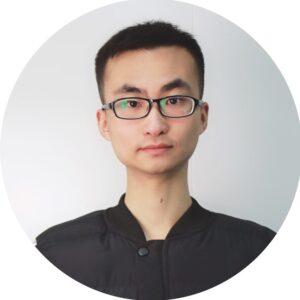
Frank
Frank graduated from the University of Shanghai for Science and Technology, majoring in optics. As a technical engineer at Crylink Company, he deeply understands crystal materials and laser components.
Related Video(s) with this Article
Related Product(s) with this Article
Related Application(s) with this Article