Introduction
As technology advances, it brings to the fore new solutions that not only solve complex problems but also redefine productivity and precision. Two such technological marvels are Solid-State Lasers and Fiber Lasers. Both have their unique characteristics and applications, yet their differences often leave people scratching their heads. In this comprehensive guide, we will delve into their unique attributes, operational principles, and their comparative analysis to gain a deeper understanding of these cutting-edge laser technologies.
Understanding Solid-State Lasers
Solid-state lasers, a cornerstone of the laser technology landscape, have a rich history that dates back to the mid-20th century. Since their inception in the 1960s, these lasers have evolved, bringing along significant developments in numerous fields, including manufacturing, medicine, and communication. To appreciate their importance and continued relevance in a world increasingly dominated by newer technologies, it is crucial to grasp the fundamental principles of their operation and understand their unique characteristics.
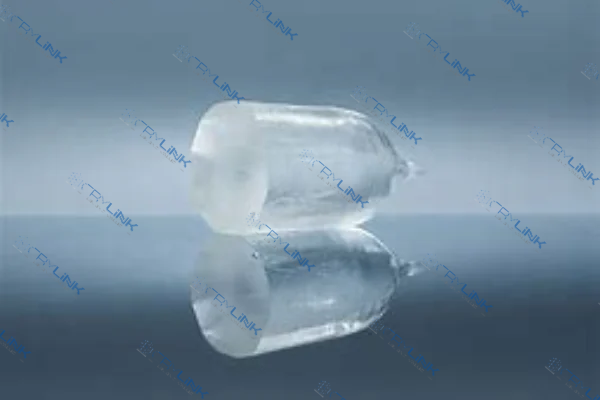
At the heart of a solid-state laser lies a solid gain medium, typically a glass or crystalline structure infused with ions. The type of ions employed can vary and are carefully chosen based on the desired properties of the resultant laser beam. Commonly used ions include neodymium (Nd), yttrium (Y), and erbium (Er), each offering distinct emission spectra and operational characteristics. These ions, when embedded in the host medium, provide the energy states necessary for the laser operation.
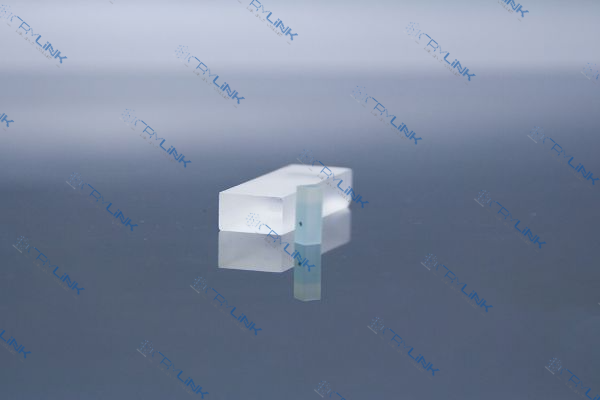
The creation of a laser beam within a solid-state laser is a multi-step process. It begins with the absorption of energy from an external light source by the doped ions in the gain medium. These external light sources are often high-intensity lamps, capable of delivering the energy needed to pump the ions to a higher energy state. Upon receiving this energy, the ions get excited and rise to a state of higher energy, a process known as population inversion.
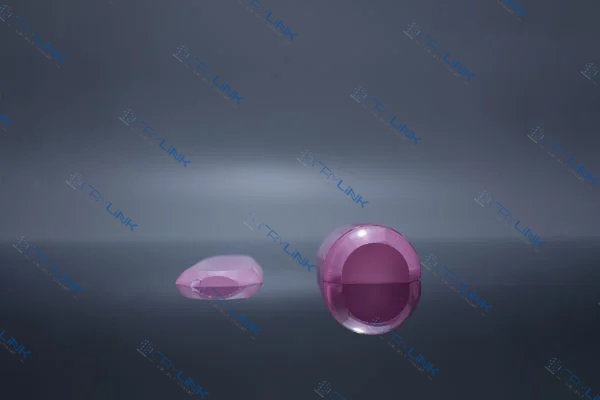
Population inversion sets the stage for what is arguably the most critical step in laser operation – the stimulated emission of radiation. When an excited ion comes across a photon that matches its energy difference between the higher and lower state, it gets stimulated to drop down to the lower energy state, emitting a photon in the process. This emitted photon, which is identical to the stimulating photon, amplifies the light within the gain medium, leading to the generation of the laser light.
However, merely generating the light within the gain medium is not enough; the light needs to be directed and contained to create a coherent and powerful laser beam. This is achieved through a resonant optical cavity. Solid-state lasers typically use a pair of mirrors placed at either end of the laser medium to form this cavity. One mirror is highly reflective, bouncing back most of the light into the medium, while the other is partially reflective, allowing a small portion of the light to escape. This setup allows the light to bounce back and forth within the medium, stimulating further emissions and amplifying the light to form the laser beam.
The operation of solid-state lasers does not occur in a vacuum; several factors, including the temperature of the gain medium, the intensity of the pumping light, and the properties of the resonant cavity, all play vital roles in the laser’s performance. Managing these factors is critical to maintaining the laser’s efficiency and reliability.
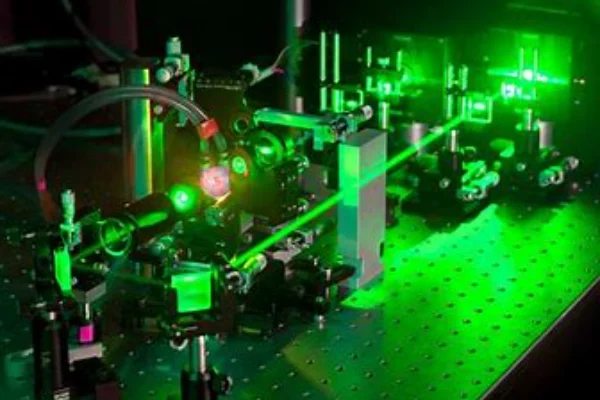
Unraveling Fiber Lasers
Fiber lasers stand as a testament to the remarkable advancements in laser technology. Their development marked a significant shift from traditional solid-state lasers, paving the way for more compact, efficient, and durable laser systems. With their unique operational mechanism and unparalleled capabilities, fiber lasers have dramatically reshaped various industries, from manufacturing and telecommunications to medicine and scientific research.
At the core of a fiber laser lies an optical fiber, a flexible, hair-thin strand of glass or plastic, known for its ability to guide light over long distances with minimal loss. This optical fiber serves as the active gain medium of the laser, the heart of the laser operation. However, unlike the undoped glass or plastic fibers used in telecommunications, the fiber in a fiber laser is doped with rare-earth elements, such as erbium or ytterbium. This doping introduces the energy states necessary for laser operation, allowing the fiber to not only guide light but also amplify it.
The operation of a fiber laser shares several similarities with that of a solid-state laser. It begins with an external light source pumping energy into the doped optical fiber. This energy excites the doped ions to higher energy states, leading to a state of population inversion, a prerequisite for laser operation. As in solid-state lasers, the next step is the stimulated emission of radiation. However, the unique structure of the optical fiber brings a new twist to this process.
In a fiber laser, the core of the fiber acts as a waveguide, ensuring the light travels along the fiber. The cladding surrounding the core reflects any light trying to escape back into the core, allowing the light to bounce back and forth within the fiber, stimulating further emissions and amplifying the light. This bouncing of light effectively lengthens the path the light travels within the gain medium, leading to significant amplification even with a short length of fiber.
One of the breakthroughs in fiber laser technology is the double-clad fiber design. A double-clad fiber has a second layer of cladding around the inner cladding, often shaped to capture and redirect the pump light into the core. This design allows the pump light to be introduced into the fiber at multiple points, ensuring more uniform pumping and enabling the fiber laser to operate at high power levels without damaging the fiber.
The fiber’s flexible nature presents another significant advantage, offering unparalleled design versatility. The fiber can be coiled, bent, and arranged in various configurations, allowing the laser to be tailored to specific applications. Furthermore, the fiber’s small size and lack of need for external mirrors reduce alignment issues common with solid-state lasers, making fiber lasers more robust and easier to maintain.
The remarkable efficiency and durability of fiber lasers are not accidental but the result of their unique design and operation. They leverage the best qualities of optical fibers – low loss, flexibility, and resilience – while mitigating their limitations through clever engineering, such as double-clad design. The result is a laser that is not only compact and versatile but also capable of delivering high power levels and superior beam quality, qualities that have propelled fiber lasers to the forefront of laser technology.
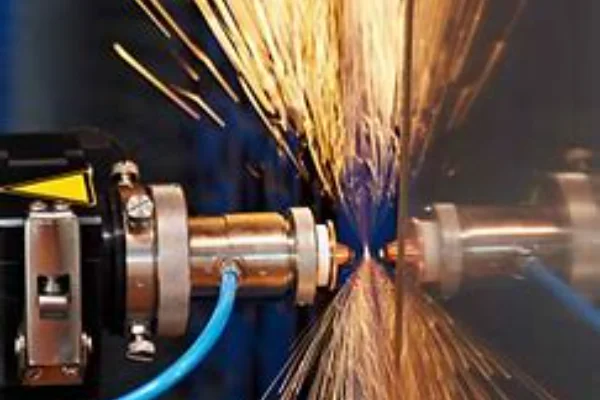
Key Differences Between Solid-State and Fiber Lasers
While both solid-state lasers and fiber lasers operate on the same basic principle of stimulated emission, they differ significantly in their design, performance, and application areas. These differences can largely be traced back to their distinct gain media: a solid glass or crystalline structure for solid-state lasers and a flexible, doped optical fiber for fiber lasers.
One of the most striking differences between solid-state and fiber lasers lies in their performance and efficiency. Fiber lasers are recognized for their exceptional electrical efficiency, thanks to the nature of the fiber optic cable, which can conduct light with minimal loss. This feature makes fiber lasers incredibly energy-efficient, often achieving efficiencies over 30%. Solid-state lasers, on the other hand, typically have lower efficiencies, which can be attributed to the higher losses in their bulkier gain media and the need for high-intensity lamps for pumping.
However, efficiency is just one aspect of performance. Another critical performance metric is beam quality, which directly impacts the laser’s effectiveness in precision applications. Here too, fiber lasers outshine their solid-state counterparts. The single-mode operation of fiber lasers delivers an incredibly high beam quality, characterized by a tight focus and minimal divergence. Solid-state lasers, while capable of delivering high-quality beams, often struggle to match the beam quality of fiber lasers, especially at higher power levels.
Despite the lower efficiency and beam quality, solid-state lasers are not without their strengths. They boast robust power scaling capabilities, making them ideal for high-power applications. Solid-state lasers can be designed to generate incredibly high power levels by increasing the size of the gain medium and the pumping power, something that is not as straightforward with fiber lasers due to the limitations of the fiber’s size and heat dissipation.
The size and maintenance requirements of the two laser types also set them apart. By virtue of their design, solid-state lasers tend to be bulkier than fiber lasers. The need for a large gain medium and external mirrors contributes to their size and weight, limiting their suitability for applications where space is at a premium.
Maintenance is another factor that favors fiber lasers over solid-state lasers. The alignment of the mirrors in a solid-state laser is critical to its operation and requires regular checks and adjustments, adding to the maintenance workload. Furthermore, solid-state lasers often require active cooling to manage the heat generated in the gain medium, which not only adds to the system’s complexity but also its maintenance needs.
Fiber lasers, in contrast, are remarkably compact and virtually maintenance-free. The small size of the fiber and the absence of external mirrors greatly reduce the alignment issues associated with solid-state lasers. Additionally, the fiber’s excellent heat dissipation capabilities often eliminate the need for active cooling, further reducing the maintenance needs.
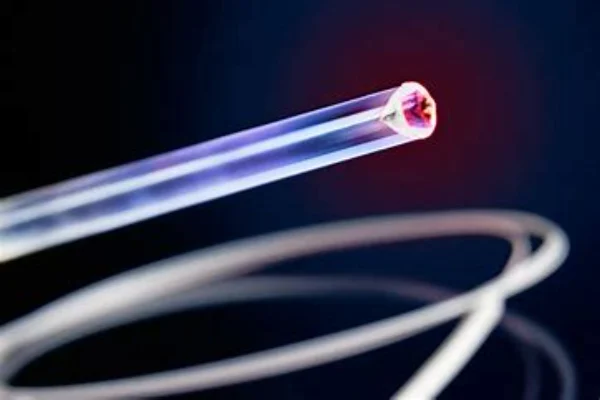
Conclusion
To conclude, both solid-state lasers and fiber lasers come with their unique strengths and weaknesses. While solid-state lasers excel in power, fiber lasers offer unparalleled efficiency and beam quality. It ultimately boils down to the specific needs of the operation. Understanding their differences is, therefore, key to harnessing their potential effectively.
FAQs
- Q1: What is the primary difference between a solid-state laser and a fiber laser?
- A1: The main difference lies in their gain medium. A solid-state laser uses a solid gain medium, such as doped glass or crystals, whereas a fiber laser uses a doped optical fiber as its gain medium.
- Q2: Where are solid-state lasers typically used?
- A2: Solid-state lasers are commonly used in high-power applications such as laser cutting, laser welding, and industrial machining.
- Q3: What makes fiber lasers more efficient than solid-state lasers?
- A3: Fiber lasers have a high electrical-to-optical conversion efficiency, superior beam quality, and their design enables efficient heat dissipation, making them more efficient than solid-state lasers.
- Q4: Are fiber lasers safer to operate than solid-state lasers?
- A4: Yes, fiber lasers are generally safer to operate because the laser light remains confined within the fiber, reducing the risk of accidental exposure.
- Q5: Which laser is more durable – solid-state or fiber laser?
- A5: Fiber lasers are considered more durable and resilient to environmental changes due to their solid-state construction and the absence of free-space optical components.
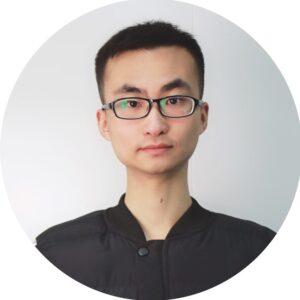
Frank
Frank graduated from the University of Shanghai for Science and Technology, majoring in optics. As a technical engineer at Crylink Company, he deeply understands crystal materials and laser components.
Related Video(s) with this Article
Related Product(s) with this Article
Related Application(s) with this Article