Introduction
In the realm of optics and laser systems, optical dispersion presents a formidable challenge. As light travels through different mediums, its speed varies, leading to the phenomenon of dispersion. This can adversely affect the performance of laser systems, especially in applications requiring high precision. Fortunately, with the advent of specific crystals, it is possible to counteract these effects and maintain pulse integrity. In this article, we delve deep into the intricacies of optical dispersion and elucidate how crystals play a pivotal role in overcoming these challenges.
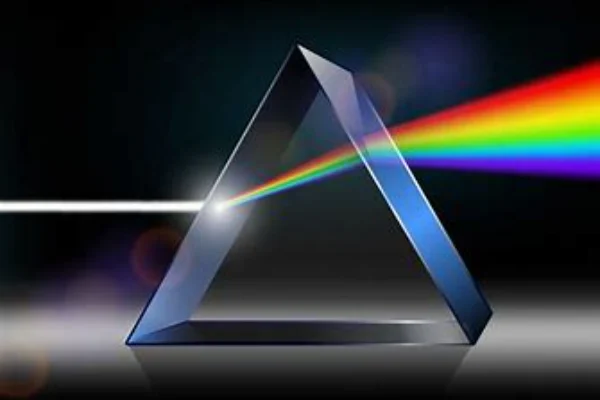
Understanding Optical Dispersion
Optical dispersion, a fundamental concept in the field of optics, plays a pivotal role in how we perceive and utilize light in various applications. At its core, optical dispersion is the variation of light’s speed as it traverses through different mediums. This variation is dependent on the light’s wavelength, meaning that different colors or wavelengths of light will travel at slightly different velocities when passing through the same medium.
Imagine a beam of white light, which is essentially a combination of multiple colors, entering a prism. As each color has its distinct wavelength, they all travel at unique speeds within the prism. This results in the light spreading out and separating into its constituent colors, creating a spectrum. This beautiful display, while mesmerizing, is a direct consequence of optical dispersion and is the reason behind phenomena like rainbows.
However, the effects of optical dispersion aren’t just limited to creating visual spectacles. In practical applications, especially in communication systems that rely on light signals, dispersion can introduce challenges. For instance, when light signals with multiple wavelengths are transmitted through optical fibers, the varying speeds of these wavelengths can lead to the signals spreading out. This can distort the transmitted information, leading to potential data loss or the need for signal correction.
The extent of dispersion is influenced by several factors. One of the primary determinants is the material through which the light is passing. Different materials have unique refractive indices, which dictate how much they can bend or slow down light. The relationship between a material’s refractive index and the light’s wavelength is intricate. For some materials, longer wavelengths might travel faster, while for others, the opposite might be true.
Temperature is another factor that can influence dispersion. As the temperature of a medium changes, its density and, consequently, its refractive index can also change. This can lead to variations in how different wavelengths of light travel through the medium.
In conclusion, optical dispersion is a complex yet fascinating phenomenon that has both aesthetic and practical implications. Understanding its nuances is crucial for fields ranging from telecommunications to photography, and its mastery can lead to innovations that harness light in novel ways.
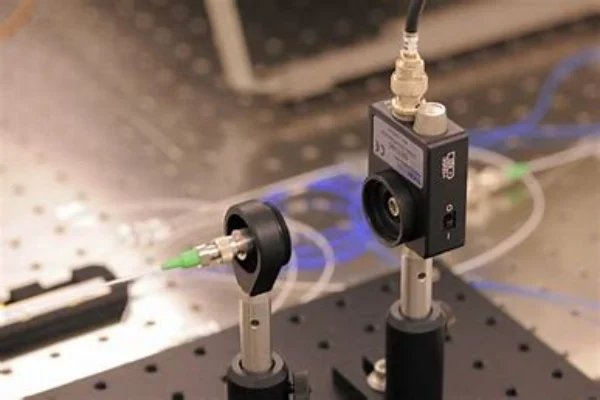
Effects of Optical Dispersion in Laser Systems
Optical dispersion in laser systems can have a profound impact on their performance and efficiency. One of the primary consequences of this phenomenon is pulse broadening. When a laser pulse, which is a short burst of light, travels through a medium that exhibits dispersion, the different wavelengths within the pulse travel at different speeds. This differential speed causes the pulse to spread out over time, increasing its duration. As a result, the energy of the pulse, which was initially concentrated over a short time, gets distributed over a longer period. This leads to a reduction in the peak intensity of the pulse, which can be detrimental in applications where high peak power is essential.
Another significant effect of optical dispersion is temporal distortions. In a laser system, a pulse is composed of various frequency components. Due to dispersion, these components can reach the detector or the end of the system at different times. This misalignment in arrival times can cause the pulse shape to be distorted, affecting the information or energy it carries. In applications like optical communication, where data is transmitted using light pulses, these distortions can lead to errors, reducing the system’s reliability and efficiency.
Furthermore, optical dispersion can introduce challenges in nonlinear optical processes. One such challenge is phase mismatch. Nonlinear processes, such as second-harmonic generation, rely on the interaction of light waves at different frequencies to produce new frequencies. For these processes to be efficient, the phases of the interacting waves need to be aligned. However, dispersion can cause a mismatch in these phases, leading to a significant reduction in the efficiency of the nonlinear process. This means that even if a system is pumped with high power, the desired output, like the second harmonic, might be weak.
In essence, while optical dispersion is a natural phenomenon, its effects on laser systems can be quite challenging. It necessitates careful design and consideration to ensure that laser systems can function optimally, especially in critical applications where precision and efficiency are paramount.
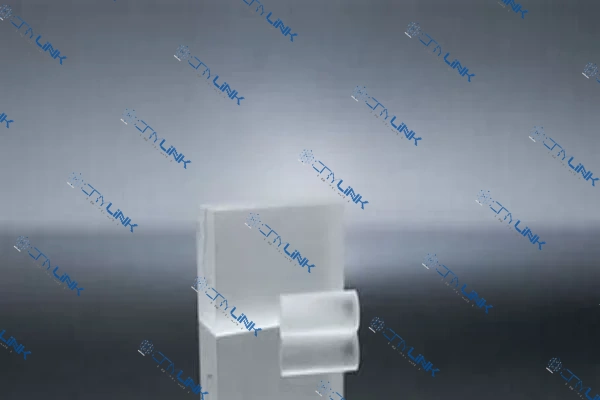
Crystals: The Solution to Dispersion Challenges
Crystals have long been recognized as powerful tools in the realm of optics, offering solutions to challenges that often seem insurmountable. Among the myriad of problems they address, dispersion in laser systems stands out as a particularly pressing issue. Fortunately, certain crystals, specifically designed for dispersion compensation, have emerged as frontrunners in tackling this challenge.
The unique properties of dispersion-compensating crystals make them invaluable in optical setups. One of the most sought-after traits in these crystals is negative group velocity dispersion. In simple terms, this means that the crystal can slow down shorter wavelengths more than longer ones, effectively reversing the effects of normal dispersion. This property allows these crystals to counterbalance the dispersion introduced by other elements in the system, ensuring that all wavelengths of a pulse remain synchronized as they travel.
Another critical property of these crystals is their high damage threshold. Laser systems, especially those used in research and industrial applications, can generate pulses with extremely high peak powers. The materials used in these systems, therefore, need to withstand these intense light bursts without getting damaged. Dispersion-compensating crystals are specially designed to endure such conditions, ensuring system longevity and reliability.
Among the plethora of crystals available for dispersion compensation, a few have distinguished themselves due to their exceptional properties. Beta Barium Borate, or BBO, is one such crystal. Its wide phase-matching bandwidth makes it versatile, suitable for a range of wavelengths. Moreover, its high damage threshold ensures it can be used in high-power systems without the risk of degradation.
Potassium Dihydrogen Phosphate, commonly known as KDP, is another crystal that has found widespread use in optical systems. Its popularity can be attributed to its large angular bandwidth, which offers flexibility in system design. Additionally, the ease with which large, high-quality KDP crystals can be grown makes them a preferred choice for many applications.
Lastly, Lithium Niobate (LiNbO3) has carved a niche for itself, primarily due to its efficiency in second-harmonic generation. This property allows it to double the frequency of incoming light, a process vital in many optical applications.
In conclusion, as the demand for high-precision, efficient laser systems grows, the role of dispersion-compensating crystals becomes ever more crucial. These crystals, with their unique properties, stand as testament to the marvels of modern material science, driving advancements in the field of optics.
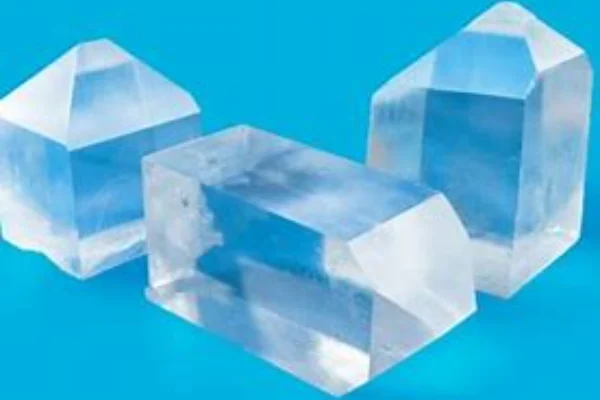
Maintaining Pulse Integrity with Crystals
In the intricate world of laser systems, maintaining pulse integrity is of paramount importance. The pulse, a brief and concentrated burst of light, carries with it information, energy, or both. Any alteration to its shape or duration can lead to inefficiencies, inaccuracies, or even system failures. Dispersion, as we’ve discussed, is a primary culprit behind such pulse deformations. However, with the advent of dispersion-compensating crystals, there’s a beacon of hope for preserving pulse integrity.
Imagine a musical note played on a piano. If this note gets distorted, it might sound off-key or stretched out, losing its original character. Similarly, in a laser system, the pulse needs to maintain its ‘note’ or shape to ensure the system’s optimal performance. Dispersion-compensating crystals act as the perfect tuning mechanism in this scenario. When a pulse, distorted due to passing through a dispersive medium, encounters one of these crystals, something remarkable happens. The crystal, with its unique properties, imparts a dispersion that is opposite in nature to the one introduced by the medium. This counteractive dispersion works to reshape the pulse, molding it back to its original form.
The strategic placement of these crystals within the laser system is crucial. Just as a skilled musician knows where to tune their instrument for the best sound, an optical engineer must know where to position these crystals for maximum effect. When placed correctly, these crystals can work wonders, not only restoring the pulse’s shape but also ensuring its duration remains unchanged. This restoration is vital for applications where timing and pulse shape are critical, such as in high-precision measurements or data transmission.
In essence, dispersion-compensating crystals serve as the guardians of pulse integrity. They stand as a testament to how understanding and harnessing the properties of materials can lead to innovative solutions, ensuring that laser systems deliver peak performance consistently.
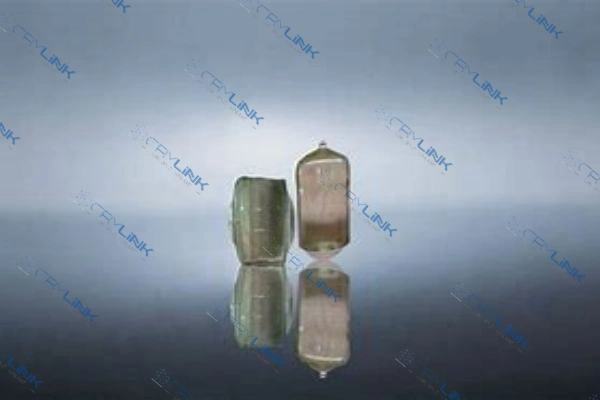
Conclusion
Optical dispersion, while a natural phenomenon, can pose significant challenges in laser systems. However, with the right understanding and the use of specific crystals, these challenges can be effectively addressed. By leveraging the unique properties of dispersion-compensating crystals, we can ensure that laser systems operate at their peak efficiency, maintaining pulse integrity and delivering optimal performance.
Frequently Asked Questions (FAQs)
- What causes optical dispersion in laser systems?
- Optical dispersion is caused by the differential speed of light of different wavelengths when passing through a medium.
- How do dispersion-compensating crystals work?
- These crystals introduce an opposite dispersion to that of the dispersive medium, effectively canceling out the effects.
- Are there any side effects of using dispersion-compensating crystals?
- When used appropriately, these crystals enhance the performance of laser systems without introducing adverse side effects.
- Can any crystal be used for dispersion compensation?
- No, only specific crystals with properties like negative group velocity dispersion and high damage threshold are suitable for this purpose.
- Is it possible to completely eliminate optical dispersion in laser systems?
- While it’s challenging to entirely eliminate dispersion, with the right crystals and system design, its effects can be significantly minimized.
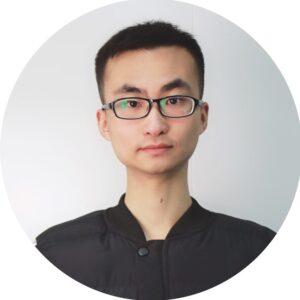
Frank
Frank graduated from the University of Shanghai for Science and Technology, majoring in optics. As a technical engineer at Crylink Company, he deeply understands crystal materials and laser components.
Related Video(s) with this Article
Related Product(s) with this Article
Related Application(s) with this Article