Introduction
In the realm of optoelectronics, the orientation of crystals plays a pivotal role in determining the performance and efficiency of devices. Crystal orientation, particularly the E-cut and Z-cut, has a profound impact on the optical properties and functionalities of devices. In this comprehensive guide, we delve deep into the significance of crystal orientation and its influence on optoelectronic devices.
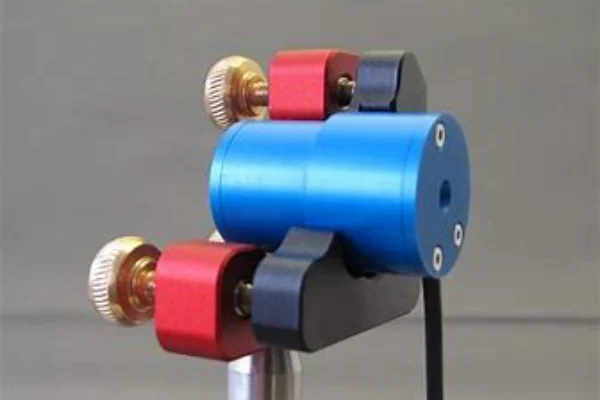
Understanding Crystal Orientation
Understanding the intricacies of crystal orientation is fundamental in the realm of materials science and optoelectronics. At its core, crystal orientation is the specific arrangement of atoms or molecules within a crystalline structure. This isn’t a random or arbitrary arrangement; instead, it follows a particular direction or pattern. The way these atoms or molecules align can have profound implications, especially when we consider the physical and optical properties of the material in question.
Take, for instance, the difference between E-cut and Z-cut crystals. The distinction might seem subtle to the untrained eye, but for those in the know, the differences are monumental and can influence the application and performance of these crystals in various devices.
E-cut crystals are specifically cut in a manner that’s perpendicular to the electric field direction. But why does this matter? In applications where the interaction with the electric field is paramount, E-cut crystals shine. Their unique orientation means they are primed to interact with electric fields in a way that other cuts might not be. This makes them invaluable in specific technological applications where such interactions are crucial for the device’s functionality.
On the other hand, Z-cut crystals have a different orientation altogether. They are cut parallel to the optical axis. This orientation is not by accident; it’s by design. Z-cut crystals are pivotal for applications that center on light propagation and interaction. Their alignment is tailored to ensure that they interact with light in a very particular manner, making them indispensable in devices where light behavior is of the essence.
But beyond the technical jargon, what does this mean in practical terms? It means that the way a crystal is oriented can dictate its behavior and, by extension, the behavior of the device it’s a part of. It’s akin to setting the foundation for a building. The way the foundation is laid will determine the building’s stability, strength, and overall performance. Similarly, the orientation of a crystal can set the stage for how it will perform in various applications.
In the ever-evolving world of technology, where the demand for more efficient and effective devices is constant, understanding elements like crystal orientation becomes paramount. It’s not just about creating devices; it’s about creating the best devices. And to do that, every component, down to the microscopic arrangement of atoms in a crystal, must be understood and optimized. In this context, the orientation of crystals, whether E-cut or Z-cut, plays a pivotal role in pushing the boundaries of what’s possible in optoelectronic devices.
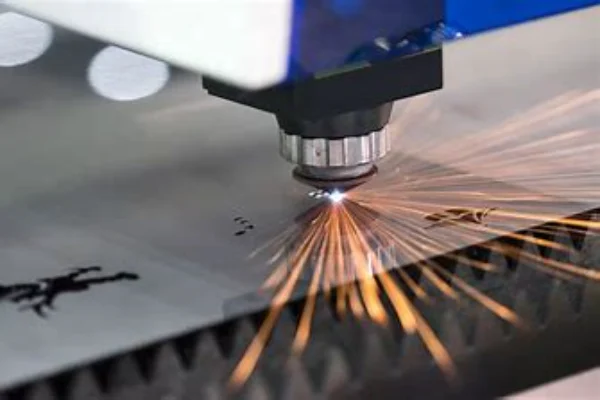
Significance of Crystal Orientation in Optoelectronic Devices
The significance of crystal orientation in optoelectronic devices cannot be overstated. At the heart of this lies the profound influence orientation has on a material’s optical properties. The way atoms or molecules align within a crystal can directly affect its refractive index, birefringence, and absorption coefficient. This is evident when we compare Z-cut and E-cut crystals. Z-cut crystals, for instance, have a distinct refractive index compared to their E-cut counterparts, leading to a diverse range of optical behaviors. Such variations can be the difference between a device working optimally and it underperforming.
Beyond just the optical properties, the orientation of a crystal plays a pivotal role in the overall performance of optoelectronic devices. A crystal that’s oriented correctly can be the catalyst for a device’s enhanced performance. Consider light-emitting diodes (LEDs), for example. The efficiency of light emission in LEDs can be significantly improved with the right crystal orientation. Similarly, in the realm of photodetectors, the sensitivity of these devices can be heightened by ensuring the crystal within is oriented correctly. Such nuances might seem minor, but in the grand scheme of device performance, they can be game-changers.
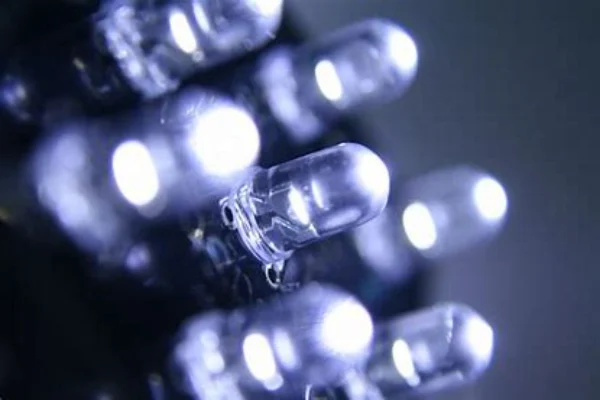
Furthermore, the versatility of crystal orientation allows for the tailoring of optoelectronic device functionalities. By tweaking the orientation, we can achieve specific outcomes. Whether it’s achieving a particular polarization state or manipulating phase matching conditions in nonlinear optical devices, the possibilities are vast. This ability to customize functionalities means that devices can be fine-tuned to serve specific purposes or to perform under certain conditions, making them more adaptable and efficient.
In essence, the orientation of crystals is akin to the tuning of a musical instrument. Just as the right tuning can produce harmonious music, the correct crystal orientation can lead to the optimal performance of optoelectronic devices. It’s a delicate balance, but when achieved, it paves the way for advancements in optoelectronic technology, driving innovation and enhancing the capabilities of devices we rely on daily.
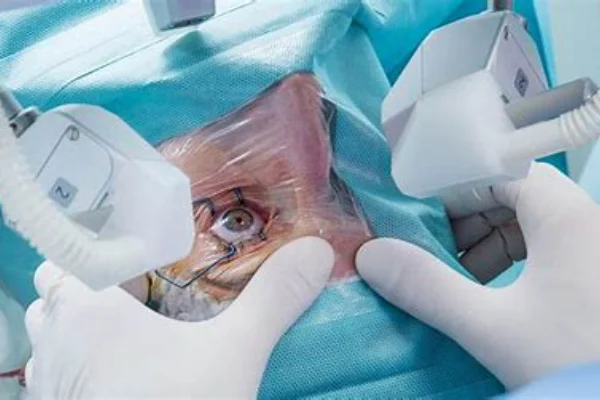
Applications Benefiting from Crystal Orientation
The realm of optoelectronics has witnessed significant advancements, and a key player in this progress is the orientation of crystals. This seemingly microscopic detail holds the power to dictate the performance, efficiency, and functionality of a range of devices. Let’s delve deeper into how different applications benefit from the meticulous selection and manipulation of crystal orientation.
Consider lasers and Light Emitting Diodes (LEDs), which are ubiquitous in today’s technological landscape. These devices rely heavily on the emission of light, and the characteristics of this emission can be influenced by the orientation of the crystal lattice within. The emission wavelength, which determines the color and type of light emitted, and the efficiency of this emission,
which dictates how much energy is converted into light, can both be optimized by selecting the right crystal orientation. For instance, a specific orientation might be chosen to produce a laser with a very precise wavelength for medical applications, or an LED that emits a particular shade of light for display screens. The beauty lies in the adaptability; by tweaking the crystal orientation, we can fine-tune these devices to serve specific needs.
Shifting our focus to photodetectors and solar cells, the story of crystal orientation continues to unfold. These devices are at the forefront of renewable energy solutions and are pivotal in converting light into electricity. The efficiency of this conversion process is heavily influenced by how the crystal within the device is oriented. The absorption of light, a critical step in the conversion process, and the subsequent generation of electron-hole pairs, which are essential for electricity generation, can be optimized with the right orientation. In simple terms, a solar cell with a correctly oriented crystal can harness more sunlight and convert it into more electricity, making it more efficient and effective.
Lastly, in the intricate world of modulators and switches, particularly electro-optic modulators, crystal orientation plays a starring role. These devices are all about the interaction between light and electric fields. The depth of modulation, which determines how much the light signal is altered, and the speed at which this modulation occurs, are both influenced by the orientation of the crystal. By ensuring the crystal is oriented in a way that maximizes its interaction with the applied electric field, we can achieve faster and more precise modulation, which is crucial for applications like high-speed data transmission.
In conclusion, the orientation of crystals is not just a minute detail; it’s a critical factor that influences the performance of a plethora of optoelectronic devices. From the lasers used in surgeries to the solar panels harnessing the sun’s energy, the meticulous selection of crystal orientation is shaping the future of technology, making devices more efficient, adaptable, and tailored to specific needs.
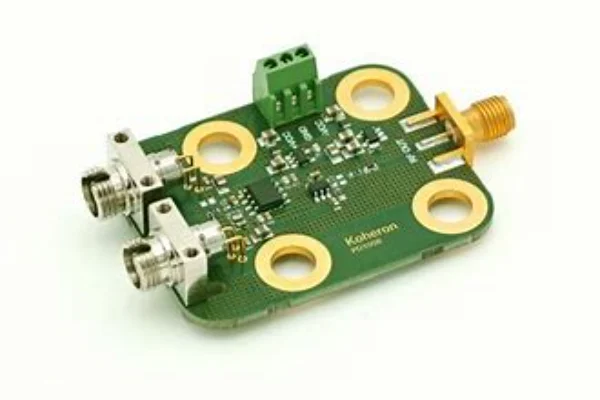
Challenges and Considerations in Crystal Orientation
While crystal orientation offers numerous advantages, it’s essential to consider certain challenges. Achieving the desired orientation requires precise cutting techniques. Moreover, the material’s inherent properties, such as its symmetry and lattice structure, can limit the orientations achievable.
Conclusion
Crystal orientation stands as a cornerstone in the domain of optoelectronics, influencing the optical properties and functionalities of devices. By understanding and harnessing the power of E-cut and Z-cut orientations, we can tailor devices to achieve specific optical behaviors, paving the way for advancements in optoelectronic technology.
FAQs
- What is the primary difference between E-cut and Z-cut crystals?
- E-cut crystals are cut perpendicular to the electric field direction, while Z-cut crystals are cut parallel to the optical axis.
- How does crystal orientation impact the efficiency of LEDs?
- The orientation of the crystal lattice can influence the emission wavelength and efficiency of LEDs, allowing for desired emission characteristics.
- Why is crystal orientation important in electro-optic modulators?
- In electro-optic modulators, the crystal’s orientation determines the interaction between the applied electric field and the propagating light, affecting the device’s performance.
- Can any crystal orientation be achieved in all materials?
- No, the material’s inherent properties, such as its symmetry and lattice structure, can limit the orientations achievable.
- How does crystal orientation influence the refractive index of a material?
- Crystal orientation can directly impact the refractive index, leading to varied optical behaviors in different orientations.
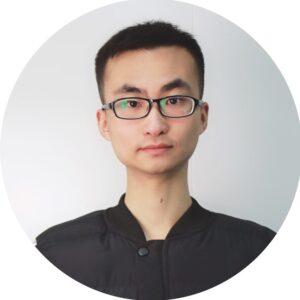
Frank
Frank graduated from the University of Shanghai for Science and Technology, majoring in optics. As a technical engineer at Crylink Company, he deeply understands crystal materials and laser components.
Related Video(s) with this Article
Related Product(s) with this Article
Related Application(s) with this Article