Introduction to Electro-Optic Modulators
Electro-Optic Modulators (EOMs) are critical components in modern communication systems. They convert electronic signals into optical signals, enabling high-speed data transmission over long distances. The heart of these devices is the electro-optic crystal, which exhibits a change in refractive index when an electric field is applied.
The Role of Crystals in Electro-Optic Modulators
The choice of crystal in an EOM significantly impacts the device’s performance. The crystal’s properties, such as its electro-optic coefficient and dielectric constant, determine the modulator’s response time and modulation efficiency.
Lithium Niobate: The Industry Standard
Lithium Niobate (LiNbO3), with its high electro-optic coefficient, has been the cornerstone of the electro-optic modulator industry for many years. This crystal has been the go-to choice for manufacturers and researchers alike, primarily due to its excellent electro-optic properties that allow for efficient conversion of electronic signals into optical ones. This conversion is the fundamental operation that enables the transmission of data over long distances at high speeds, a necessity in our increasingly connected world.
However, despite its widespread use and apparent advantages, LiNbO3 is not without its drawbacks. One of the most significant issues associated with this crystal is photorefractive damage. This phenomenon occurs when high-intensity light alters the crystal’s refractive index, leading to a distortion of the transmitted light signal. Over time, this can degrade the performance of the modulator, leading to a decrease in the quality of data transmission.
Photorefractive damage is particularly problematic in high-power or high-intensity applications, where the light intensity can quickly induce changes in the crystal’s refractive index. This issue is further exacerbated in environments with fluctuating temperatures, as temperature changes can enhance the photorefractive effect.
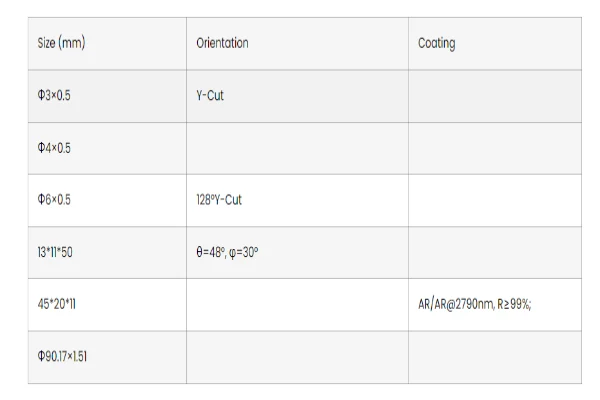
Furthermore, LiNbO3 crystals are typically grown in large, high-quality single crystals, a process that can be both time-consuming and costly. This adds to the overall cost of manufacturing electro-optic modulators, making it a less attractive option, especially for applications requiring a large number of modulators.
Despite these challenges, LiNbO3 remains a popular choice due to its high electro-optic coefficient. However, the industry is increasingly recognizing the need for alternatives. Researchers are now exploring other materials that can offer similar or even superior performance, without the drawbacks associated with LiNbO3. These new materials could potentially revolutionize the field of electro-optic modulators, paving the way for more efficient, reliable, and cost-effective communication systems.
In conclusion, while Lithium Niobate has served the industry well for many years, its limitations, particularly concerning photorefractive damage, highlight the need for ongoing research and development in this field. The quest for the perfect electro-optic crystal continues, with the promise of exciting advancements on the horizon.
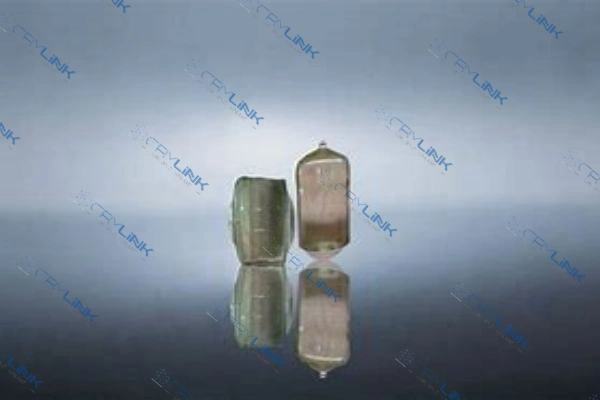
Exploring Alternative Crystals
To overcome the limitations of LiNbO3, researchers have been exploring alternative crystals. These include Barium Titanate (BaTiO3) and Potassium Titanyl Phosphate (KTP), which offer improved performance and resistance to photorefractive damage.
Barium Titanate (BaTiO3)
Barium Titanate (BaTiO3) is another crystal that has been gaining attention in the field of electro-optic modulators. This ferroelectric crystal is known for its high electro-optic coefficient, a property that makes it a strong contender for use in EOMs. The high electro-optic coefficient allows for efficient modulation of light, which is crucial for high-speed data transmission in communication systems.
However, similar to Lithium Niobate, BaTiO3 also exhibits a strong photorefractive effect. This effect can lead to changes in the refractive index of the crystal when exposed to intense light, which can distort the transmitted light signal and degrade the performance of the modulator over time. This is a significant challenge that needs to be addressed when considering BaTiO3 for use in EOMs.
Interestingly, researchers have found ways to mitigate the photorefractive effect in BaTiO3. One such method is through doping, where small amounts of another element are introduced into the crystal. This process can alter the crystal’s properties and reduce its photorefractive effect. For instance, doping BaTiO3 with elements like manganese or cobalt has been shown to significantly decrease its photorefractive effect.
Another approach to mitigating the photorefractive effect in BaTiO3 is operating at higher wavelengths. The photorefractive effect is less pronounced at higher wavelengths, meaning that the refractive index changes less and the light signal distortion is minimized. This makes BaTiO3 a suitable candidate for applications that operate at these wavelengths.
Despite these challenges and the methods to overcome them, BaTiO3 has several advantages that make it a promising material for EOMs. Its high electro-optic coefficient and the ability to mitigate its photorefractive effect make it a potential alternative to Lithium Niobate. Moreover, BaTiO3 crystals are easier and less costly to grow than LiNbO3 crystals, making them a more cost-effective option for EOM manufacturing.
In conclusion, while BaTiO3 does present some challenges, its high electro-optic coefficient and the potential to overcome its photorefractive effect make it a promising candidate for future EOMs. As research continues in this field, we can expect to see further advancements and potentially new materials that could revolutionize the industry.
Potassium Titanyl Phosphate (KTP)
Potassium Titanyl Phosphate, commonly known as KTP, is another crystal that has been gaining traction in the field of electro-optic modulators. KTP is known for its robustness and high damage threshold, making it a durable choice for various applications. These characteristics are particularly beneficial in high-power applications, where the crystal needs to withstand intense light without suffering damage.
One of the key advantages of KTP is its excellent thermal stability. This property is crucial in maintaining the performance of the modulator over time. In many applications, the modulator can heat up due to the high power of the light passing through it. If a crystal is not thermally stable, this heat can cause changes in its properties, leading to a decrease in the modulator’s performance. However, with its excellent thermal stability, KTP can maintain its properties even at high temperatures, ensuring consistent performance.
However, it’s important to note that KTP has a lower electro-optic coefficient than LiNbO3. This means that it is less efficient at converting electronic signals into optical signals. While this might seem like a significant disadvantage, it’s worth noting that the electro-optic coefficient is just one of many factors that determine a modulator’s performance. Other factors, such as the crystal’s thermal stability and damage threshold, can also play a significant role.
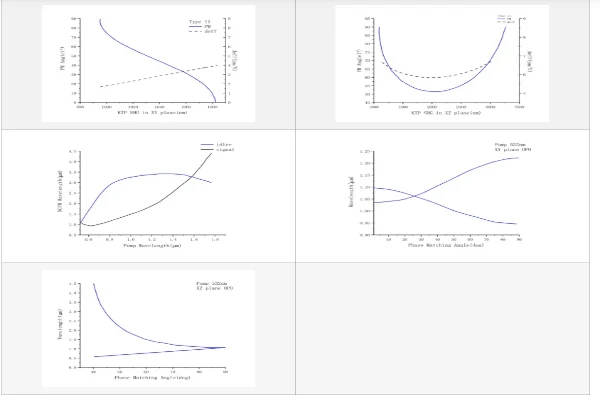
Despite its lower electro-optic coefficient, KTP’s robustness and thermal stability make it a promising candidate for high-power applications. These applications often require crystals that can withstand high power levels without suffering damage or significant changes in their properties. With its high damage threshold and excellent thermal stability, KTP fits this requirement well.
In addition to its robustness and thermal stability, KTP also has other advantages. For instance, it is transparent over a wide range of wavelengths, making it suitable for a variety of applications. It also has a high nonlinear coefficient, which makes it efficient for frequency conversion processes, such as second harmonic generation.
In conclusion, while KTP may have a lower electro-optic coefficient than LiNbO3, its robustness, high damage threshold, and excellent thermal stability make it a promising candidate for high-power applications. As research continues in this field, we can expect to see further advancements in the use of KTP and other crystals in electro-optic modulators.
-crylink.webp)
The Future of Electro-Optic Modulators
The future of electro-optic modulators is a dynamic landscape, shaped by the relentless pursuit of technological advancement. As we continue to push the boundaries of communication systems, the need for faster, more efficient modulators becomes increasingly paramount. This demand fuels the ongoing exploration for the ideal crystal for EOMs, a quest that is as challenging as it is exciting.
The evolution of communication systems is not a linear path, but rather a complex interplay of various factors. The need for speed, efficiency, and reliability must be balanced against practical considerations such as cost, availability of materials, and ease of integration into existing systems. As such, the search for the ideal crystal is not merely about finding a material with the highest electro-optic coefficient, but rather a holistic approach that considers all these factors.
In this endeavor, the exploration of new materials plays a crucial role. Emerging materials, such as novel ferroelectric crystals or engineered structures like photonic crystals, hold the promise of unprecedented performance. At the same time, refining existing materials, such as improving the thermal stability of LiNbO3 or mitigating the photorefractive effect in BaTiO3, can lead to significant enhancements in modulator performance.
In conclusion, the future of electro-optic modulators is a journey of continuous exploration and refinement. As we delve deeper into the world of crystals and their potential applications in EOMs, we are not only paving the way for the next generation of communication technology, but also contributing to the broader advancement of science and technology.
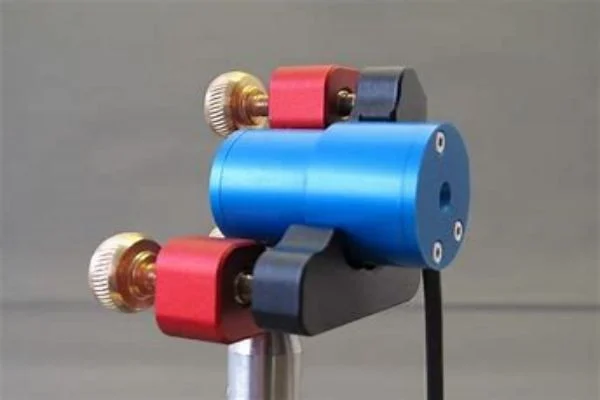
Conclusion
In the realm of communication systems, the exploration of crystals for electro-optic modulators is a dynamic and exciting field. As we move beyond lasers and delve deeper into the world of electro-optics, the potential for innovation is vast. The future of communication may well be shaped by the crystals we are just beginning to understand.
Frequently Asked Questions
- 1. What is an Electro-Optic Modulator?
- An Electro-Optic Modulator is a device that converts electronic signals into optical signals.
- 2. Why are crystals important in Electro-Optic Modulators?
- Crystals are the core component of EOMs. Their properties determine the modulator’s performance.
- 3. What is the industry standard crystal for EOMs?
- Lithium Niobate (LiNbO3) is currently the industry standard due to its high electro-optic coefficient.
- 4. What are some alternative crystals being explored?
- Alternative crystals include Barium Titanate (BaTiO3) and Potassium Titanyl Phosphate (KTP).
- 5. What is the future of Electro-Optic Modulators?
- The future of EOMs lies in the exploration of new materials and the refinement of existing ones to meet the evolving demands of communication systems.
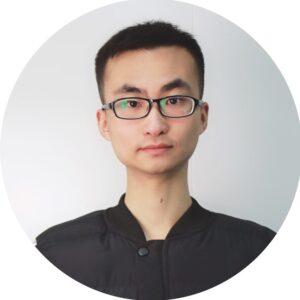
Frank
Frank graduated from the University of Shanghai for Science and Technology, majoring in optics. As a technical engineer at Crylink Company, he deeply understands crystal materials and laser components.
Related Video(s) with this Article
Related Product(s) with this Article
Related Application(s) with this Article